Ketamine: A Review for Clinicians
Abstract
A growing series of clinical trials and case series now suggest that ketamine—originally used as an anesthetic agent—potentially offers an exciting new treatment option for severe depression. Increasing numbers of studies show that ketamine can provide prompt relief for many depressed patients, including those with severe treatment-refractory depression. Although the effects of a single treatment are commonly short-lived, multiple infusion protocols may offer sustained relief. The uniquely rapid onset of antidepressant action raises the potential for ketamine use in a variety of clinical situations, including the prevention or shortening of hospital stays, the treatment of acute suicidal ideation, and the facilitation of medication crossovers. Ketamine, in combination with other multimodal treatment approaches, including psychotherapy, may further augment response effect and duration. Promises of efficacy have led to increasingly unbridled use to treat a variety of psychiatric disorders, with diverse approaches and treatment environments, despite inadequate data demonstrating the true clinical efficacy and safety of the various protocols or a thorough understanding of mechanisms of action. This article briefly reviews the history of ketamine’s development as a potential antidepressant, current hypotheses related to its mechanisms of action, and existing evidence for its safety and efficacy with a focus on clinicians’ interests.
There is clear evidence highlighting the need for new and more effective treatments for depression. Up to one third of patients do not respond to typical treatment strategies. When effective, responses can take weeks to months, and even then relapse rates remain unacceptably high (1). These shortcomings are further amplified by the significant morbidity and mortality associated with depression and their impact on individuals, families and society. To date, nearly all U.S. Food and Drug Administration (FDA)-approved medications to treat depression have developed from the same monoamine-based hypotheses dominating the field since the 1960s, with little success in identifying other effective pharmacological approaches. Thus considered, there is deserved enthusiasm about emerging evidence suggesting that ketamine, a medication initially approved by the FDA in 1970 as an anesthetic agent, can induce rapid-onset antidepressant effects in a substantial proportion of patients.
This combination of unmet need for more effective and rapidly acting antidepressant treatments, with mounting evidence demonstrating ketamine’s rapid antidepressant properties, has led to exponential growth of its off-label use (2). The use of ketamine is outpacing the published data demonstrating the efficacy and safety of the treatment. In this article, we attempt to provide a brief overview of the events leading to the discovery of ketamine’s antidepressant effects, review the current data surrounding the efficacy and safety of the drug, and outline a rational path forward for practicing clinicians.
The Pathophysiology of Depression: The Glutamate Theory
The monoamine theory of depression has historically dominated our pharmacologic approaches. Studies examining the physiological consequences of chronic stress have provided the field with new insights into additional pathogenic and pathophysiological mechanisms of depression. The brain’s response to acute stress may serve an adaptive role in survival; however, exposure to chronic stress may lead to long-term synaptic injury and dysfunction (3). Chronic and severe stress are associated with dysregulation of the hippocampal-pituitary-adrenal axis, maladaptive changes in neurotransmitter signaling, alterations in inflammatory pathways, and dysregulation of neurotrophic factor signaling—all capable of altering neuroplasticity and producing maladaptive changes in brain structure and function.
A growing series of studies have demonstrated the critical role of glutamate in modulating neuroplasticity and suggest that stress-induced changes in this system’s function may contribute to the neurobiology of mood disorders (3, 4). Work dating back to the early 1990s demonstrated specific changes in the function of the glutamatergic N-methyl-d-aspartate (NMDA) receptor following periods of stress exposure and that drugs acting as NMDA antagonists could produce antidepressant-like effects in rodent models (5). Chronic stress also affects glutamatergic α-amino-3-hydroxy-5-methyl-4-isoxazolepropionic acid (AMPA) receptors, closely associated with the regulation of neurotrophic factors, including brain-derived neurotrophic factor (BDNF) and other intracellular signaling pathways that modulate local protein synthesis, synaptic spine numbers, and synaptic function. Altered levels of synaptic plasticity and dysfunction of circuits within the prefrontal cortex (PFC) and hippocampus in rodent models have been linked to common symptoms of depression. Correlated with such rodent studies, patients with depression frequently demonstrate decreased brain volumes in regions of the PFC and hippocampus (6), related to episode duration and severity (7–9), and may be reversible with effective treatment (10).
Although the monoamine-based antidepressants alter synaptic plasticity (11, 12), the effects are subtle and seen only after prolonged exposure. This model has been used to explain the delayed effects of classical antidepressants and also serves as an impetus to identify more rapidly acting treatments.
Ketamine Pharmacology
Ketamine acts as a high-affinity, noncompetitive, open-channel NMDA receptor blocker. The NMDA receptor is an ionotropic (ligand-gated transmembrane ion channel) receptor within the glutamatergic neurotransmitter system, along with AMPA and kainate receptors. Glutamate serves as the major excitatory neurotransmitter in the central nervous system. Its roles in neuroplasticity, cognition, memory, and learning are thought to be mediated in large part through modulation of the NMDA receptors, which are present ubiquitously throughout the brain (13). As a consequence of the NMDA receptor’s central role in brain function, the receptor is highly regulated by a variety of physiological factors, which results in a highly complex receptor pharmacology.
When functioning correctly, glutamate binds and causes the NMDA receptor channel to open, allowing calcium ions to cross the neuronal membrane, which only occurs with the cobinding of glycine or serine (or both) in the correct local pH (Figure 1A). These strict physiological conditions serve to maintain optimal signal-to-noise detection within the nervous system and prevent neuronal excitotoxity. Ketamine blocks the NMDA receptor channel, preventing ion influx and attenuating the generation of action potentials in neurons containing NMDA receptors.
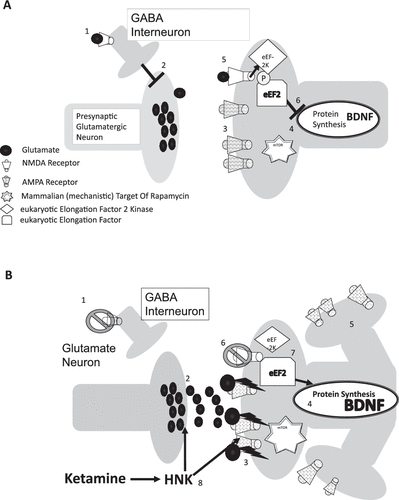
FIGURE 1. Models of Ketamine’s Mechanism of Actiona
aA: Glutamate surge model of the absence of ketamine. Under normal physiological conditions, activation of NMDA receptors on inhibitory GABAergic interneurons (1) tonically inhibits release of presynaptic glutamate (2), resulting in minimal activation of postsynaptic AMPA receptors (3), low levels of BDNF expression, and mTOR activation to drive baseline local protein synthesis. Under the desuppression-of-translation model, low levels of spontaneous stimulation of postsynaptic NMDA receptors cause an activation of elongation factor 2 kinase (5) and subsequent phosphorylation of elongation factor 2, resulting in inhibition of local protein synthesis, including BDNF expression (6). B: Glutamate surge model of the presence of subanesthetic doses of ketamine. This model proposes that subanesthetic doses of ketamine selectively block NMDA receptor function on inhibitory GABAergic interneurons (1), thus causing a surge in glutamate release (2) and an increased phasic activation of the postsynaptic AMPA receptors (3) that results in increased local protein and BDNF expression (4). This is believed to result in further AMPA activation and local increases in neuroplasticity and synaptogenesis (5). The desuppression model suggests that subanesthetic doses of ketamine are selectively blocking the function of NMDA receptors at rest, responding to spontaneous glutamate release (6). This response results in decreased activation of elongation factor 2 kinase, dephosphorylation and activation of elongation factor 2 (7), and increased levels of local protein synthesis, including BDNF (4). This would result in an increased insertion of AMPA receptors and generate the same downstream effects as proposed above on BDNF, local protein synthesis and synaptogenesis (3–5). More recently, data generated with hydroxynorketamine and other novel compounds suggest that NMDA receptor blockade may not be necessary to achieve the cellular response associated with ketamine. Instead, this model proposes that the effects of these drugs are produced by either non-NMDA–mediated stimulated release of presynaptic glutamate (2) or direct activation of the postsynaptic AMPA receptors (3) that then initiate the same downstream cascade of events leading to increased neuroplasticity (4 and 5). Abbreviations: BDNF, brain-derived neurotrophic factor; mTOR, mechanistic target of rapamycin; eEF2, eukaryotic elongation factor 2; eEF-2K, eukaryotic elongation factor 2 kinase; HNK, hydroxynorketamine
While ketamine’s effects on the NMDA receptor are believed to be the central mechanism mediating the more immediate hypnotic and analgesic effects of the drug, there are several other molecular actions that may help mediate its effects on consciousness, pain, cognition, and possibly depression. Examples of these mechanisms include an inhibition of the hyperpolarization-activated cationic channel (HCN1) (14), activation of the nitric oxide-cyclic guanosine-monophosphate (cGMP) pathway (15), modulation of opioid receptors (16), and inhibition of nicotinic acetylcholine receptors (17). In addition, ketamine also has reported downstream effects on monoaminergic (18) and neuropeptide signaling (19), as well as inflammation (20).
Ketamine has a notably short half-life, ranging from 100 to 200 minutes (as reported at the 36th Meeting of the WHO Expert Committee on Drug Dependence in Geneva, Switzerland, June 16–20, 2014), and is completely metabolized within a few hours of dosing, suggesting that antidepressant mechanisms are tied to downstream effects of the drug. Hypotheses regarding ketamine’s more immediate pharmacological effects have been largely based on rodent microdialysis and electrophysiological studies that suggested that subanesthetic doses of ketamine and other NMDA-receptor antagonists have selective activity on NMDA receptors located on inhibitory GABAergic interneurons, especially those in the medial prefrontal cortex and the hippocampus (21–23). Blocking NMDA receptor-related GABAergic interneuron activity, thereby releasing tonic inhibition of glutaminergic neurons, is thought to generate a selective increase in glutamate release, or “glutamate surge,” in specific brain regions (Figure 1B). Several lines of research now suggest that this glutamate surge is associated with ketamine’s antidepressant-like response in rodent models (3, 24, 25); however, the data remain far from conclusive.
The next step in the proposed cascade is believed to be the region-specific activation of postsynaptic AMPA receptors. Several preclinical studies, using AMPA-receptor antagonists such as NBQX to block the antidepressant-like effects of ketamine and other glutamate-modulating drugs, suggest that this is a necessary element of ketamine’s antidepressant mechanism (26–28). The need for AMPA activation is one of the most consistent findings in the preclinical literature and has been reported in multiple studies of drugs associated with a rapid onset of antidepressant activity (29–33). There remains, however, some discrepancy on whether effects on glutamate release are necessary (32) and whether action at the NMDA receptor is a necessary component of the drug’s antidepressant action at all (29).
Believed to be a consequence of the increased activation of AMPA glutamate receptors, rapid increases in regional brain metabolism have been shown in several studies with subanesthetic ketamine administration in rodents and humans (34–37), most prominent in several regions closely associated with mood and depressive symptomology. Emerging evidence now suggests that there are rapid neuroplastic changes in several of these brain regions following ketamine administration in rodent models in the form of increased dendritic spine densities (3), electrophysiological measures of connectivity (38), and imaging measures of functional connectivity (39). These structural and cytoarchitectual changes are believed to be correlates to several recent findings that suggest dramatic changes in brain connectivity in humans, downstream from the immediate pharmacological effects (25, 40, 41).
Clinical Perspectives
Inspired by the emerging preclinical data outlined earlier, the first study to specifically assess ketamine’s utility in treating major depressive disorder was performed by Krystal and colleagues at Yale University in the late 1990s (42). The dose, 0.5 mg/kg infused over 40 minutes, was chosen on the basis of previous studies by this group showing that this dose had significant effects on cognition and perception but still allowed the subject to remain awake enough to answer questions and perform simple tasks (43). The large magnitude and rapid onset of antidepressant effects observed in the study were surprising. Notably, although the study generated immediate interest in the glutamatergic system as a novel target for antidepressant development, it took another 6 years before these findings were replicated (44). Since this time, there have been numerous small replication studies yielding largely consistent findings regarding the rapid antidepressant effects of a single subanesthetic dose of ketamine (45, 46). These results, combined with the tremendous unmet need for more effective treatments, have led to a significant increase in the off-label use of ketamine for the treatment of depression and other psychiatric disorders (2). The remaining sections of this article outline and highlight the many unresolved questions and concerns related to this treatment.
Dosing
There has been astonishingly little research attempting to identify optimal dosing strategies for ketamine. As noted earlier, the original study demonstrating ketamine’s antidepressant actions (42) used a dose of 0.5 mg/kg intravenously (i.v.) delivered over 40 minutes, based on the drug’s transient effects on cognition and perception, without consideration of optimal dosing for antidepressant effects. Interestingly, evidence now suggests that ketamine has a unique inverted U-shaped dose-response relationship in rodent models, which is most effective at moderate subanesthetic doses (24). However, remarkably, there have been relatively few dose-optimization studies performed until recently.
A few small pilot studies have attempted assess ketamine dose titration, but these studies are very challenging to interpret, given small sample sizes and complicated study designs (47–49). Two larger controlled studies examining the effects of various subanesthetic doses of ketamine have recently been completed. The first found a significant dose-related effect of ketamine, whereby a 0.5-mg/kg dose appeared more efficacious than a 0.2-mg/kg dose of ketamine in treating more severely depressed patients (50). The second, a multisite study funded through the National Institute for Mental Health’s Rapidly-Acting Treatments for Treatment-Resistant Depression mechanism, attempted to explore the antidepressant effects of ketamine across a range of doses (0.1, 0.2, 0.5, and 1 mg/kg compared with a midazolam control) in a between-subjects design (presented in 2017 at the annual meeting of the American Society of Clinical Psychopharmacology). The study clearly demonstrated the superior effect of ketamine compared with midazolam, but because of the limited power of the study, it was unable to statistically identify an optimal dose. However, the 0.5-mg/kg dose numerically gave the largest and most consistent signal of antidepressant efficacy across multiple outcome measures. In light of the limited high-quality data that are currently available, it is difficult to provide clear guidance on optimal dosing, but increasing evidence supports that a dose-response relationship of some sort does exist. A survey of providers suggests that a significant proportion of clinicians offering the treatment are continuing to use the 0.5-mg/kg dosing i.v. over 40 minutes (2).
The question of long-term effectiveness remains a challenge in assessing the clinical value of ketamine. The large majority of the randomized controlled studies performed to date have used a single dose of the drug. While the antidepressant effects of single dosing may be sustained for days to weeks in many patients, near-complete relapse of symptoms is common when patients are followed for extended periods of time (51), promoting the exploration of approaches capable of sustaining or prolonging the effects. There are now several case series and clinical reports suggesting that the antidepressant effects can be sustained for periods of several months with repeated administration of ketamine (51–54), which we have also seen in our clinical practice (55). However, relatively little high-quality–controlled data have been published to demonstrate the sustained effectiveness of the drug. A single randomized placebo-controlled study has evaluated the effects of the frequency of repeated ketamine dosing (56). The findings from this study of 67 patients suggested that a dosing of twice per week was just as effective as thrice per week, and both regimens showed large sustained effects for the two weeks of randomized dosing compared with a saline placebo. In an open-label continuation phase of this study, the large majority of the patients maintained their response for at least an additional two weeks with ongoing treatment. The results from the recent survey of providers suggest that the large majority of clinicians are administering doses of the medication either two or three times a week to initiate treatment (2); however, there is no clear consensus on the optimal dosing strategy.
The optimal route of administration is also unclear. The original studies used dosing i.v., and the large majority of published studies to date have continued to use this route. However, alternative routes have been explored. An early report by Lapidus et al. (57) showed the potential use of the intranasal administration of ketamine for major depressive disorder. The ease of use and rapid absorption of the drug makes this route appealing, but problems controlling bioavailability (58) and tolerability (59) do present challenges. More recently, intranasal administration of esketamine has been shown to be effective in a randomized, placebo-controlled study (60), and a large phase-III program is under way to evaluate the efficacy and safety of intranasal esketamine that should provide results in the near future. Other studies have explored administration via subcutaneous injections (47) and oral administration (61, 62). However, the volume and quality of the published data on these routes of administration remain extremely limited.
In summary, the effects of a single ketamine infusion are, unfortunately, often short-lived for most patients, which has increasingly led clinicians to provide series of infusions to prolong response. However, data supporting this specific regimen remain relatively limited and even less so for maintenance strategies. To date, we are not aware of any published controlled trials extending beyond 1 month of ongoing treatment. However, the growing number of case series and open-label reports do suggest that the antidepressant effects can be sustained with periodic repeated dosings for some patients. The risks of both single and repeated dosing are described later in the article.
Other Indications
Ketamine is now frequently being prescribed for off-label use as a treatment for major depression disorder. A few studies have specifically assessed the efficacy and safety of ketamine for bipolar depression (63–66). These relatively small proof-of-concept studies suggest an efficacy similar to that for its use for major depressive disorders but that may be associated with a shorter duration of antidepressant effect. Notably, the majority of these studies treated patients with bipolar disorder who were concurrently taking a mood stabilizer, either lithium or valproate.
On the basis of the proposed mechanisms of action, there is growing interest in ketamine’s potential utility in the treatment of posttraumatic stress disorder (PTSD) (67). Although several case series and a single clinical trial assessing ketamine’s efficacy in PTSD (68) suggest some clinical benefit, there are little quality data to evaluate at this time. A large study sponsored by the U.S. Department of Veterans Affairs and the U.S. Department of Defense (NCT02655692) is currently under way, with plans to evaluate the efficacy of ketamine in nearly 200 military personnel with PTSD over the next few years.
Based in part on patient reports, there is also increased interest in ketamine for treating anxiety disorders. Glue and colleagues observed what appeared to be dose-related improvements in 10 out of 12 nondepressed patients with generalized anxiety disorder, social anxiety, or both in an open-label study with subcutaneous, ascending, weekly dosing of ketamine (69). Another small study of 18 patients evaluated a single infusion of ketamine compared with saline placebo for social anxiety in a sequential design. The authors reported a meaningful clinical response in 33% of the patients after ketamine administration, whereas there were no responders after placebo administration (70). Two studies showed mixed results with obsessive-compulsive disorder (OCD): Rodriguez and colleagues found a positive effect of ketamine in patients with OCD when comparing a single infusion of ketamine to saline within a double-blind crossover trial (71); however, a previous small, open-label study by Bloch and colleagues found no meaningful clinical benefit (72).
As may be expected, based on the proposed effects of ketamine on neuroplasticity, interest in ketamine has spread well beyond the standard psychiatric indications. Ongoing areas of interest range from chronic pain (73) to Parkinson’s disease (74), to Rett’s syndrome (75). However, the bottom line is that there are few studies with limited sample sizes to suggest clinical benefit in any of these indications at the present time, possibly beyond pain management.
Another worthy question is whether ketamine used in combination with electroconvulsive therapy (ECT) for depression can produce enhanced benefits. Researchers have hoped to find a synergistic role for ketamine in combination with ECT for major depressive disorder, either for augmentation of antidepressant effects or cognitive protection. Unfortunately, although complicated to evaluate, neither have been consistently demonstrated in study populations (76). Further studies are necessary to better evaluate the benefit of ketamine as the primary anesthetic agent, as well as the use of ketamine administered with prolonged infusion protocols for these purposes.
Last, there is an increasing amount of higher quality data to support ketamine’s effects on suicidal ideation (77–80). The existing studies suggest a rapid onset of antidepressant effects associated with ketamine treatment that may, to some extent, be independent of the drug’s effects on other symptoms of depression. An individual patient-level meta-analysis containing most of these data found ketamine to produce a moderate-to-large effect size (Cohen’s d=0.48–0.85) for the reduction of suicidal ideation for up to one week in duration after a single dosing (80). Interestingly, the effect was seen even after adjusting for concurrent reductions in depressive symptoms. A recently completed study with esketamine showed marked improvement in depressive symptoms and suicidal ideation in patients with major depression expressing imminent, severe suicidal ideation, behavior, or both (81). Interestingly, these benefits were seen in addition to very good response rates to placebo combined with standard-of-care treatments of these patients.
Risks of Use
Understanding the risks associated with ketamine use is an essential component of making any risk-benefit assessment. Although ketamine has well-characterized transient effects on cardiovascular, cognitive, and perceptual functions (43, 82), there are significantly less published data on the safety of repeated administrations. The acute effects on cardiovascular function suggest the need for a thorough pretreatment physical examination to identify those at potential risk of harm and close monitoring during treatment. It also continues to limit the environment in which ketamine can be safely administered (83). Although a recent report of 66 patients who received a total of 684 ketamine infusions over 40 min at 0.5 mg/kg for the treatment of depression at Emory University found blood pressure changes that were only considered “small, well tolerated and clinically insignificant” (84), there have been rare elevations in blood pressure high enough to cause concern for individuals at risk for cardiovascular complications in several reports. Considering the limited quality data available at this time, we continue to strongly recommend treatment environments capable of cardiac monitoring with medically trained nurses and physicians until more high-quality safety data can be reviewed.
As noted earlier, ketamine frequently has significant transient effects on cognition, perception, and anxiety, even when administered to the healthy volunteers. Patients, not uncommonly, have some level of distress and anxiety during or immediately after the treatment. These are typically short-lived and resolve soon after infusion completion. Nevertheless, these can be disturbing to some patients and may interfere with their desire to continue treatment. For these reasons, clinicians should observe patients during and shortly after infusions until these symptoms resolve completely and should be prepared to provide appropriate behavioral management measures if needed.
Longer term risks of repeated ketamine use are largely extrapolated from a small population of ketamine abusers and animal studies, with understandable limitations. In retrospective observational studies, ketamine abusers have been reported to suffer from chronic cystitis, hepatotoxicity, and gall bladder pathology, along with psychiatric symptoms of impaired cognition and persistent dissociative effects (85). To date, it is not possible to determine the rates of such symptoms at the doses and frequencies most commonly used to treat depression. Perhaps the good news is that, to our knowledge, there is no clear evidence of a causative association between the doses and frequencies discussed here for the treatment of major depressive disorder with urinary complications. However, patients treated with maintenance ketamine should clearly be closely monitored until more data are collected. Last, there is the risk of fostering ketamine abuse or dependence in patients. Possibly because of its dissociative, stimulant, or analgesic effects, or its activity at opioid receptors, ketamine is a common drug of abuse in many regions of the world. Although we do not believe that this necessarily prohibits consideration of the drug for the treatment for appropriate patients, patients should be carefully screened for a history of substance use disorders and be followed closely for any signs of substance use disorder during the treatment series. At present, take-home ketamine dosing is not recommended because of the risks associated with the transient effects and the risk of abuse and diversion (83).
Conclusions
Growing evidence supports ketamine’s robust and prompt antidepressant effects, and recent findings suggest that the antidepressant effects may be extended for some period of time with continued treatment. However, there are many unanswered questions and concerns related to the drug’s practical use in the clinical management of patients. There are ongoing efforts to understand the mechanisms underlying ketamine’s effects on mood. Although the more immediate pharmacological effects of the drug remain somewhat in question, there is growing convergent evidence that it does involve some level of neuroplastic change that extends well beyond the presence of the drug in body. This is of specific interest clinically, as it suggests that there may be a unique window of opportunity to engage other psychosocial treatments while the brain is more “malleable” (86). Although studies related to optimal dose, route, and frequency of administration are now beginning to be published, there are extremely little high-quality data on which to base clinical opinions. At present, there is little doubt that the greatest amount of evidence exists for the 0.5 mg/kg dose provided i.v. over 40 min. However, future studies are likely to provide us with alternative dosing strategies with equivalent or possibly improved risk:benefit ratios. There is also a dearth of published data on the longer term benefits of the treatment. Several groups are now starting to present their clinical findings with relatively large numbers of patients receiving longer term ketamine treatment at meetings. Although the quality of these reports is not comparable to that of large, well-controlled clinical trials, it will be important to the field to see these reports in peer-reviewed publications at some point in the near future. The completion of several large phase-III trials with intranasal esketamine will provide results in the next year. This large database of both long and shorter term treatments will be directly applicable to the use of esketamine but will continue to leave questions regarding the use of racemic ketamine, especially delivered by other routes, doses, and frequencies.
There are extremely little published data related to the use of ketamine for disorders other than major depressive disorder at this time. The use of ketamine for these indications is admittedly intriguing, and possibly even promising, but we all should be aware of the limited published data on hand to support its use at this time. Looking to the future, we strongly recommend the formation of a centralized registry and database of ketamine use to allow for aggregated high-quality data collection, safety reports, and regulatory purposes. There are many inherent ethical challenges accompanying the use of an agent such as ketamine, but we believe that, with cautious rational use and development, it could serve a central role in the future of psychopharmacology.
1 : Acute and longer-term outcomes in depressed outpatients requiring one or several treatment steps: a STAR*D report. Am J Psychiatry 2006; 163:1905–1917Crossref, Google Scholar
2 : A survey of the clinical, off-label use of ketamine as a treatment for psychiatric disorders. Am J Psychiatry 2017; 174:695–696Crossref, Google Scholar
3 : Synaptic plasticity and depression: new insights from stress and rapid-acting antidepressants. Nat Med 2016; 22:238–249Crossref, Google Scholar
4 : The stressed synapse: the impact of stress and glucocorticoids on glutamate transmission. Nat Rev Neurosci 2011; 13:22–37Crossref, Google Scholar
5 : Functional antagonists at the NMDA receptor complex exhibit antidepressant actions. Eur J Pharmacol 1990; 185:1–10Crossref, Google Scholar
6 : Structural neuroimaging studies in major depressive disorder. Meta-analysis and comparison with bipolar disorder. Arch Gen Psychiatry 2011; 68:675–690Crossref, Google Scholar
7 : Association of brain cortical changes with relapse in patients with major depressive disorder. JAMA Psychiatry 2018 (Epub March 28, 2018). doi:
8 : Depression duration but not age predicts hippocampal volume loss in medically healthy women with recurrent major depression. J Neurosci 1999; 19:5034–5043Crossref, Google Scholar
9 : Effects of cumulative illness severity on hippocampal gray matter volume in major depression: a voxel-based morphometry study. Psychol Med 2018 (Epub February 8, 2018). doi:
10 : Hippocampal volume, memory, and cortisol status in major depressive disorder: effects of treatment. Biol Psychiatry 2004; 56:101–112Crossref, Google Scholar
11 : Synaptic dysfunction in depression: potential therapeutic targets. Science 2012; 338:68–72Crossref, Google Scholar
12 : Chronic fluoxetine administration enhances synaptic plasticity and increases functional dynamics in hippocampal CA3-CA1 synapses. Neuropharmacology 2017; 126:250–256Crossref, Google Scholar
13 : NMDA receptors in the central nervous system. Methods Mol Biol 2017; 1677:1–80Crossref, Google Scholar
14 : Forebrain HCN1 channels contribute to hypnotic actions of ketamine. Anesthesiology 2013; 118:785–795Crossref, Google Scholar
15 : Ketamine activates the L-arginine/nitric oxide/cyclic guanosine monophosphate pathway to induce peripheral antinociception in rats. Anesth Analg 2011; 113:1254–1259Crossref, Google Scholar
16 : Activation of N-methyl-D-aspartate receptor attenuates acute responsiveness of delta-opioid receptors. Mol Pharmacol 1997; 51:583–587Crossref, Google Scholar
17 : Subunit-dependent inhibition of human neuronal nicotinic acetylcholine receptors and other ligand-gated ion channels by dissociative anesthetics ketamine and dizocilpine. Anesthesiology 2000; 92:1144–1153Crossref, Google Scholar
18 : Impact of subanesthetic doses of ketamine on AMPA-mediated responses in rats: An in vivo electrophysiological study on monoaminergic and glutamatergic neurons. J Psychopharmacol 2015; 29:792–801Crossref, Google Scholar
19 : Ketamine strengthens CRF-activated amygdala inputs to basal dendrites in mPFC layer V pyramidal cells in the prelimbic but not infralimbic subregion, a key suppressor of stress responses. Neuropsychopharmacology 2015; 40:2066–2075Crossref, Google Scholar
20 : Ketamine and peripheral inflammation. CNS Neurosci Ther 2013; 19:403–410Crossref, Google Scholar
21 : NMDA receptor hypofunction produces opposite effects on prefrontal cortex interneurons and pyramidal neurons. J Neurosci 2007; 27:11496–11500Crossref, Google Scholar
22 : Activation of glutamatergic neurotransmission by ketamine: a novel step in the pathway from NMDA receptor blockade to dopaminergic and cognitive disruptions associated with the prefrontal cortex. J Neurosci 1997; 17:2921–2927Crossref, Google Scholar
23 : Regulation of glutamate efflux by excitatory amino acid receptors: evidence for tonic inhibitory and phasic excitatory regulation. J Pharmacol Exp Ther 1995; 274:1209–1215Google Scholar
24 : Transiently increased glutamate cycling in rat PFC is associated with rapid onset of antidepressant-like effects. Mol Psychiatry 2017; 22:120–126Crossref, Google Scholar
25 : Prefrontal connectivity and glutamate transmission: relevance to depression pathophysiology and ketamine treatment. Biol Psychiatry Cogn Neurosci Neuroimaging 2017; 2:566–574Crossref, Google Scholar
26 : Cellular mechanisms underlying the antidepressant effects of ketamine: role of alpha-amino-3-hydroxy-5-methylisoxazole-4-propionic acid receptors. Biol Psychiatry 2008; 63:349–352Crossref, Google Scholar
27 : mTOR-dependent synapse formation underlies the rapid antidepressant effects of NMDA antagonists. Science 2010; 329:959–964Crossref, Google Scholar
28 : Requirement of AMPA receptor stimulation for the sustained antidepressant activity of ketamine and LY341495 during the forced swim test in rats. Behav Brain Res 2014; 271:111–115Crossref, Google Scholar
29 : NMDAR inhibition-independent antidepressant actions of ketamine metabolites. Nature 2016; 533:481–486Crossref, Google Scholar
30 : The antidepressant effects of an mGlu2/3 receptor antagonist and ketamine require AMPA receptor stimulation in the mPFC and subsequent activation of the 5-HT neurons in the DRN. Neuropsychopharmacology 2016; 41:1046–1056Crossref, Google Scholar
31 : Scopolamine rapidly increases mammalian target of rapamycin complex 1 signaling, synaptogenesis, and antidepressant behavioral responses. Biol Psychiatry 2013; 74:742–749Crossref, Google Scholar
32 : NMDA receptor blockade at rest triggers rapid behavioural antidepressant responses. Nature 2011; 475:91–95Crossref, Google Scholar
33 : Convergent mechanisms underlying rapid antidepressant action. CNS Drugs 2018; 32:197–227Crossref, Google Scholar
34 : Metabolic mapping of the rat brain after subanesthetic doses of ketamine: potential relevance to schizophrenia. Brain Res 1998; 787:181–190Crossref, Google Scholar
35 : Effects of ketamine, MK-801, and amphetamine on regional brain 2-deoxyglucose uptake in freely moving mice. Neuropsychopharmacology 2000; 22:400–412Crossref, Google Scholar
36 : Neural correlates of rapid antidepressant response to ketamine in bipolar disorder. Bipolar Disord 2014; 16:119–128Crossref, Google Scholar
37 : Metabolic hyperfrontality and psychopathology in the ketamine model of psychosis using positron emission tomography (PET) and [18F]fluorodeoxyglucose (FDG). Eur Neuropsychopharmacol 1997; 7:9–24Crossref, Google Scholar
38 : Differential effects of an NR2B NAM and ketamine on synaptic potentiation and gamma synchrony: relevance to rapid-onset antidepressant efficacy. Neuropsychopharmacology 2016; 41:1486–1494Crossref, Google Scholar
39 : Antagonism at the NR2B subunit of NMDA receptors induces increased connectivity of the prefrontal and subcortical regions regulating reward behavior. Psychopharmacology (Berl) 2018; 235:1055–1068Crossref, Google Scholar
40 : Ketamine decreases resting state functional network connectivity in healthy subjects: implications for antidepressant drug action. PLoS One 2012; 7:e44799Crossref, Google Scholar
41 : Default mode connectivity in major depressive disorder measured up to 10 days after ketamine administration. Biol Psychiatry 2018 (Epub February 15, 2018). doi:
42 : Antidepressant effects of ketamine in depressed patients. Biol Psychiatry 2000; 47:351–354Crossref, Google Scholar
43 : Subanesthetic effects of the noncompetitive NMDA antagonist, ketamine, in humans. Psychotomimetic, perceptual, cognitive, and neuroendocrine responses. Arch Gen Psychiatry 1994; 51:199–214Crossref, Google Scholar
44 : A randomized trial of an N-methyl-D-aspartate antagonist in treatment-resistant major depression. Arch Gen Psychiatry 2006; 63:856–864Crossref, Google Scholar
45 : Ketamine and other NMDA antagonists: early clinical rrials and possible mechanisms in depression. Am J Psychiatry 2015; 172:950–966Crossref, Google Scholar
46 : Ketamine and other glutamate receptor modulators for depression in adults. Cochrane Database Syst Rev 2015; (9):CD011612Google Scholar
47 : Placebo-controlled pilot trial testing dose titration and intravenous, intramuscular and subcutaneous routes for ketamine in depression. Acta Psychiatr Scand 2016; 134:48–56Crossref, Google Scholar
48 : Rapid and sustained reductions in current suicidal ideation following repeated doses of intravenous ketamine: secondary analysis of an open-label study. J Clin Psychiatry 2016; 77:e719–e725Crossref, Google Scholar
49 : Ketamine augmentation for outpatients with treatment-resistant depression: Preliminary evidence for two-step intravenous dose escalation. Aust N Z J Psychiatry 2017; 51:55–64Crossref, Google Scholar
50 : Dose-related effects of adjunctive ketamine in Taiwanese patients with treatment-resistant depression. Neuropsychopharmacology 2017; 42:2482–2492Crossref, Google Scholar
51 : Rapid and longer-term antidepressant effects of repeated ketamine infusions in treatment-resistant major depression. Biol Psychiatry 2013; 74:250–256Crossref, Google Scholar
52 : The effect of repeated ketamine infusion over facial emotion recognition in treatment-resistant depression: a preliminary report. J Neuropsychiatry Clin Neurosci 2015; 27:362–364Crossref, Google Scholar
53 : Ketamine infusions for treatment resistant depression: a series of 28 patients treated weekly or twice weekly in an ECT clinic. J Psychopharmacol 2014; 28:536–544Crossref, Google Scholar
54 : On the safety and benefits of repeated intravenous injections of ketamine for depression. Biol Psychiatry 2012; 72:e11–e12Crossref, Google Scholar
55 Acute and longer-term outcomes using ketamine as a clinical treatment at the Yale Psychiatric Hospital. Journal of Clinical Psychiatry. In pressGoogle Scholar
56 : A double-blind, randomized, placebo-controlled, dose-frequency study of intravenous ketamine in patients with treatment-resistant depression. Am J Psychiatry 2016; 173:816–826Crossref, Google Scholar
57 : A randomized controlled trial of intranasal ketamine in major depressive disorder. Biol Psychiatry 2014; 76:970–976Crossref, Google Scholar
58 : Ketamine and norketamine plasma concentrations after i.v., nasal and rectal administration in children. Br J Anaesth 1996; 77:203–207Crossref, Google Scholar
59 : Repeated intranasal ketamine for treatment-resistant depression - the way to go? Results from a pilot randomised controlled trial. J Psychopharmacol 2018; 32:397–407Crossref, Google Scholar
60 : Efficacy and safety of intranasal esketamine adjunctive to oral antidepressant therapy in treatment-resistant depression: a randomized clinical trial. JAMA Psychiatry 2018; 75:139–148Crossref, Google Scholar
61 : Oral ketamine for the rapid treatment of depression and anxiety in patients receiving hospice care. J Palliat Med 2010; 13:903–908Crossref, Google Scholar
62 : Oral ketamine in treatment-resistant depression: a clinical effectiveness case series. J Clin Psychopharmacol 2017; 37:464–467Crossref, Google Scholar
63 : A randomized add-on trial of an N-methyl-D-aspartate antagonist in treatment-resistant bipolar depression. Arch Gen Psychiatry 2010; 67:793–802Crossref, Google Scholar
64 : Replication of ketamine’s antidepressant efficacy in bipolar depression: a randomized controlled add-on trial. Biol Psychiatry 2012; 71:939–946Crossref, Google Scholar
65 : Ketamine versus midazolam in bipolar depression with suicidal thoughts: A pilot midazolam-controlled randomized clinical trial. Bipolar Disord 2017; 19:176–183Crossref, Google Scholar
66 : Ketamine augmentation rapidly improves depression scores in inpatients with treatment-resistant bipolar depression. Int J Psychiatry Clin Pract 2017; 21:99–103Crossref, Google Scholar
67 : Synaptic loss and the pathophysiology of PTSD: implications for ketamine as a prototype novel therapeutic. Curr Psychiatry Rep 2017; 19:74Crossref, Google Scholar
68 : Efficacy of intravenous ketamine for treatment of chronic posttraumatic stress disorder: a randomized clinical trial. JAMA Psychiatry 2014; 71:681–688Crossref, Google Scholar
69 : Ketamine’s dose-related effects on anxiety symptoms in patients with treatment refractory anxiety disorders. J Psychopharmacol 2017; 31:1302–1305Crossref, Google Scholar
70 : Ketamine for social anxiety disorder: a randomized, placebo-controlled crossover trial. Neuropsychopharmacology 2018; 43:325–333Crossref, Google Scholar
71 : Randomized controlled crossover trial of ketamine in obsessive-compulsive disorder: proof-of-concept. Neuropsychopharmacology 2013; 38:2475–2483Crossref, Google Scholar
72 : Effects of ketamine in treatment-refractory obsessive-compulsive disorder. Biol Psychiatry 2012; 72:964–970Crossref, Google Scholar
73 : Ketamine for chronic non-cancer pain: A meta-analysis and trial sequential analysis of randomized controlled trials. Eur J Pain 2018; 22:632–646Crossref, Google Scholar
74 : Case reports showing a long-term effect of subanesthetic ketamine infusion in reducing L-DOPA-induced dyskinesias. Case Rep Neurol 2016; 8:53–58Crossref, Google Scholar
75 : N-methyl-D-aspartate receptors, ketamine, and Rett syndrome: something special on the road to treatments? Biol Psychiatry 2016; 79:710–712Crossref, Google Scholar
76 : Adjunctive ketamine in electroconvulsive therapy: updated systematic review and meta-analysis. Br J Psychiatry 2017; 210:403–407Crossref, Google Scholar
77 : Effects of intravenous ketamine on explicit and implicit measures of suicidality in treatment-resistant depression. Biol Psychiatry 2009; 66:522–526Crossref, Google Scholar
78 : Ketamine for rapid reduction of suicidal ideation: a randomized controlled trial. Psychol Med 2015; 45:3571–3580Crossref, Google Scholar
79 : Ketamine for rapid reduction of suicidal thoughts in major depression: a midazolam-controlled randomized clinical trial. Am J Psychiatry 2018; 175:327–335Crossref, Google Scholar
80 : The effect of a single dose of intravenous ketamine on suicidal ideation: a systematic review and individual participant data meta-analysis. Am J Psychiatry 2018; 175:150–158Crossref, Google Scholar
81 : Efficacy and safety of intranasal esketamine for the rapid reduction of symptoms of depression and suicidality in patients at imminent risk for suicide: results of a double-blind, randomized, placebo-controlled study. Am J Psychiatry 2018 (Epub April 16, 2018). doi:
82 : Factors influencing the cardiovascular response to subanesthetic ketamine: a randomized, placebo-controlled trial. Int J Neuropsychopharmacol 2017; 20:909–918Crossref, Google Scholar
83 : A consensus statement on the use of ketamine in the treatment of mood disorders. JAMA Psychiatry 2017; 74:399–405Crossref, Google Scholar
84 : Blood pressure safety of subanesthetic ketamine for depression: A report on 684 infusions. J Affect Disord 2018 (Epub February 21, 2018). doi:
85 : A review of ketamine abuse and diversion. Depress Anxiety 2016; 33:718–727Crossref, Google Scholar
86 : Cognitive behavior therapy may sustain antidepressant effects of intravenous ketamine in treatment-resistant depression. Psychother Psychosom 2017; 86:162–167Crossref, Google Scholar