Understanding Emotion Regulation in Borderline Personality Disorder: Contributions of Neuroimaging
Borderline personality disorder (BPD) is a multidimensional, heterogeneous condition. Several maladaptive traits characterize patients with this “cluster B” personality disorder, including impulsive aggression, affective lability, self-injury, and identity diffusion. They often maintain volatile relationships. This volatility causes significant social and occupational impairment and yields a lifetime suicide mortality rate of almost 10%—a rate 50 times higher than in the general population (3). Several recent publications review the diagnosis, prognosis, pathophysiology, and current treatment strategies for this debilitating condition (3–5). BPD afflicts 1% to 2% of the general population, up to 10% of psychiatric outpatients, and up to 20% of psychiatric inpatients. Clinicians diagnose it more often in women. A recent study of more than 40 twin pairs with BPD reported a concordance rate of 35% for monozygotics and almost 7% for dizygotics (6).
Many patients with BPD suffer from comorbid psychiatric conditions. A study that included 59 patients with BPD found that all but one had a concurrent axis I disorder, and almost 70% had three or more (7). Sixty-one percent of the patients met criteria for major depressive disorder (MDD), 35% for posttraumatic stress disorder (PTSD), 29% for panic disorder with agoraphobia, 20% for a somatoform disorder, and 13% for a substance use disorder. Another study found that of 240 patients with BPD, almost 40% were diagnosed with at least one mood disorder (8). Specifically, 31% met criteria for MDD, 16% for dysthymia, 9% for bipolar I, and 4% for bipolar II.
Neural circuitry of emotion regulation
One common denominator to the various clinical phenotypes of BPD appears to be dysfunctional regulation of emotions. According to Linehan (9) individuals with BPD are characterized by high sensitivity to emotional stimuli, high emotional intensity, and slow return of emotional arousal to baseline. This theory is supported by a number of studies that indicate higher affective lability and higher affective intensity in subjects with BPD, as compared to individuals with other personality disorders (10, 11). Emotion regulation involves the processing, amplification, maintenance, and attenuation of emotions generated from internal and/or environmental stimuli. Davidson et al. (12) give an excellent overview of the present understanding of dysfunction in the neural circuitry of emotion regulation as it relates to impulsive aggression, a common feature of BPD. Evidence suggests that threatening or aversive stimuli activate the amygdala, which in turn activates the anterior cingulate and prefrontal cortices. While the anterior cingulate may play a role in the cognitive evaluation/processing of mood and affect regulation, it has also been implicated in responses to conflict. The orbital prefrontal cortex appears to inhibit impulsive aggression by regulating the amygdala. Related prefrontal regions (Figure 2, blue) include the dorsolateral prefrontal cortex (Figure 2, yellow), which may integrate emotions with cognition, and the ventromedial prefrontal cortex, which may involve general processing of emotions. Of note is that while some consider the ventromedial and orbital prefrontal cortices to be distinct regions (Figure 2, yellow and orange), they are often grouped as orbital prefrontal cortex in the psychiatric literature (13). In the psychiatric literature the anterior cingulate region includes not only the gyrus (Figure 2, dark pink) but also the adjacent medial prefrontal cortex (Figure 2, light pink).
Prefrontal serotonergic neurotransmission may play a role in modulating amygdala-driven emotional behavior (12). A variety of data suggest that dysfunctional serotonergic regulation predisposes individuals to the emotional disinhibition and impulsive aggression responsible for many of the volatile behaviors seen in BPD. Recent studies investigating the neurophysiological and neuroanatomical components of emotion regulation in BPD have used a number of electrophysiological, structural, and functional modalities. While these studies have been few in number and mostly unreplicated, they suggest that dysfunction in the neural circuitry of emotion regulation plays an integral role in BPD.
Electrophysiological studies
A recent focus for electrophysiological work in BPD has involved exploration of the relationship between BPD and MDD using sleep electroencephalography (EEG). While many of the initial sleep EEG studies did not differentiate between patients with and without comorbid MDD, more recent studies have taken this factor into account. These studies have evaluated several sleep parameters, including those of sleep continuity, non-REM sleep duration, and REM duration and latency. Both patients with BPD alone and those with MDD alone exhibited increased sleep latency and decreased total sleep time in three studies but not in a fourth (14–17). Two studies found decreased REM latency in both groups (14, 16). Increased REM density may also be present (16, 18), and the differences from normal may be greater in MDD than BPD (16). The relationship between BPD and MDD therefore remains unclear. It has been suggested that some of these parameters may serve as markers of predilection to mood disorders (14, 16). Whether there is a common biological origin for these two disorders is an open question.
EEG has also been used to study the electrophysiological mechanisms of self-injurious behavior in BPD. Interestingly, it has been reported that about one-half of these patients deny feeling pain during self-inflicted injury (19). Some patients with depression also report decreased pain sensitivity. Given the high comorbidity between MDD and BPD, pain perception and mood regulation may be neurophysiologically correlated. To test this hypothesis, Russ et al. (20) compared power spectrum analysis of EEG in patients with BPD who do not feel pain during self-injury (n=19) to those who do (n=22), as well as to patients with MDD (n=15) and to controls. Participants were asked to place one hand in a 10°C water bath and verbally rate their level of pain every 15 seconds for 4 minutes. The study found that in patients with BPD who denied feeling pain, theta power significantly and inversely correlated with reported pain both at baseline and during the cold water bath. There was a similar trend for patients with BPD who did report pain, though this was not statistically significant. The authors commented that in other studies, some theta activity localizes to the anterior cingulate cortex, a region that has been associated with depersonalization (19). They suggested that this association may correlate with decreased pain sensitivity, which in turn may play a role in mitigating noxious stimuli. The study also found that theta power directly correlated with the number of dissociative experiences resulting from the stimuli, providing further evidence that pain perception and mood regulation could be neuroanatomically and neurochemically related.
Structural imaging studies
Initial structural imaging studies using computed tomography (CT) revealed no volumetric or asymmetric abnormalities in patients with BPD (21, 22), other than a narrower third ventricle in healthy women and in those with BPD (23). The authors questioned the significance of this finding, however, as it could be attributable to females in general.
Subsequent structural studies have used magnetic resonance imaging (MRI) to evaluate BPD. Lyoo et al. (24) measured cortical and ventricular volumes in patients with BPD (n=25) and found 6.2% smaller frontal lobe volumes compared to control individuals. This study excluded patients with additional axis I or II diagnoses, which may render the results unrepresentative of BPD as a whole, given the high prevalence of comorbid disorders. Further, the study did not control for brain size.
Subcortical structures have also been studied in patients with BPD. Driessen et al. (25) measured hippocampal and amygdala volumes in patients with BPD reporting childhood physical or sexual abuse (n=21), which are common in this disorder. While the study found no difference in whole brain volume between patients with BPD and controls, the patients had 16% smaller hippocampal volumes. A similar trend was found in the amygdala (8%), though this reduction was not statistically significant. These results concur with data from patients with PTSD from childhood abuse, suggesting that this decrease may correlate with traumatic experience rather than with BPD per se (26). Driessen et al. found no volume differences when they separated their patients with BPD by presence of PTSD. Of note is that the authors did not differentiate between patients who had an abusive background versus those who did not, nor did they consider comorbid MDD, which has also been associated with reductions in hippocampal volume (27).
Functional imaging studies
In the following discussion, locations within the prefrontal cortex have been translated into three divisions most familiar to the psychiatric community (Figure 2) (13). In some instances, the description used within the study is included for completeness.
Functional MRI (fMRI) is a technique that localizes brain activity by imaging the influence of blood concentrations of deoxyhemoglobin, which alters signal through magnetic field changes. A recent fMRI study evaluated affective processing of women with BPD (n=6) and no comorbid psychiatric illness or substance abuse while they viewed slides of emotionally aversive or neutral scenes (28). Increased activity in the amygdala (6/6), fusiform gyrus (6/6), orbital prefrontal region (4/6), and anterior cingulate region (3/6, referred to as the medial prefrontal gyrus) was found in the patients compared to controls (Figure 3). Both patients and controls showed activation of the temporo-occipital cortex. The authors noted that increased activation of the amygdala has also been reported in PTSD and obsessive-compulsive disorder (OCD) and may reflect the intense and prolonged emotions seen in BPD. Increased activation of the fusiform gyrus, which processes complex visual features such as facial expression, may be attributable to influence from the amygdala. The orbital prefrontal region directly connects to the amygdala and may modulate amygdala-driven emotional behavior (12). Enhanced prefrontal activation may therefore reflect cortical processing of the increased amygdala activity.
Proton magnetic resonance spectroscopy ([1H]MRS) provides a measure of neuronal function. This technique generates signal by quantifying specified metabolites and plotting them on a spectrum. These metabolites include N-acetylaspartate (NAA, a marker of neuronal viability), choline (Cho, a neuronal membrane constituent), and creatinine (Cr, used as a standard due to its stable level). Signal may be expressed as a ratio of NAA or Cho to Cr or as an absolute quantification of metabolite concentration.
A pilot [1H]MRS study in patients with BPD (n=12) and no comorbid psychiatric illness assessed NAA concentration in the left dorsolateral prefrontal cortex and left striatum (29). Compared to controls, patients with BPD showed a significant (19%) reduction in absolute NAA concentration in the dorsolateral prefrontal region. Striatal levels were also decreased, but the difference was not significant, and ratios of NAA/Cr and Cho/Cr were not significantly changed, emphasizing the value of obtaining absolute quantification of metabolites.
Among the most widely used imaging techniques to measure brain activity is positron emission tomography (PET). This technique measures the signal of a radioactive tracer, such as [18F]-fluorodeoxyglucose (FDG), and represents it on a tomographic map. FDG is a glucose analog that is taken up into cells, including neurons, in the same manner as glucose. FDG cannot be metabolized, so it is trapped and thereby provides a measure of regional glucose metabolism. Various axis I disorders have demonstrated metabolic abnormalities, notably in the frontal lobes (30). In some studies, patients with OCD or panic disorder have demonstrated hypermetabolism compared to controls. In others, patients with mood disorders, alcoholism, or schizophrenia have shown hypometabolism.
At least six studies have reported data on resting FDG uptake in patients with BPD. Among the first was De la Fuente et al. (31), who studied FDG uptake specifically in the temporal lobes of patients with BPD (n=10). This region was selected to evaluate the theory that BPD may involve underlying epileptiform activity, as both patients with BPD and those with complex partial seizures share some common symptoms such as impulsivity. The study revealed no focal regions of asymmetry that would indicate an epileptic etiology, arguing against this theory.
Five studies measured resting FDG uptake throughout the brain in patients with BPD. Siever et al. (2) found no difference in uptake between patients with BPD and controls. Three studies reported decreased uptake relative to controls in the dorsolateral prefrontal region (30, 32, 33). Both increased and decreased uptake have been reported in the medial and ventrolateral prefrontal regions (30, 32–34). One study reported decreased uptake in the orbital prefrontal region (32, 33). Another found that decreased FDG uptake in this area correlated with a greater history of aggression (30). These findings support the theory that the orbital prefrontal region may modulate aggression through the inhibition of limbic regions (i.e., orbital prefrontal dysfunction results in limbic disinhibition).
Two studies reported differences in subcortical areas. De la Fuente et al. (32) found decreased uptake in the basal ganglia (caudate, lenticular nuclei, and ventral striatum) and thalamus. The anterior cingulate relays projections from higher-order sensory areas to other regions of the prefrontal cortex and basal ganglia, which is thought to enable emotions to affect motor planning. The basal ganglia are involved in the control of motor and emotional behavior, and the thalamus connects these nuclei to the cerebral cortex. The authors noted that hypometabolism of the thalamus has also been shown in bipolar depression, OCD, and schizophrenia. Hypometabolism of the basal ganglia has been shown in unipolar and bipolar depression, generalized anxiety disorder, OCD, schizophrenia, and alcohol abuse. One limitation of this study is that it included patients with BPD who had past comorbid MDD and/or past substance abuse. Jeungling et al. (34), using statistical parametric mapping rather than regions-of-interest, found decreased uptake in the hippocampus but not the basal ganglia or thalamus. The authors noted that this might be due to decreased hippocampal volume, a possibility that requires further study.
A variety of studies have linked reduced serotonergic activity with impulsivity and depressive symptoms, which are common in BPD (see review in Soloff et al. [33]). Two preliminary studies used d,l-fenfluramine (FEN) activated FDG to investigate possible serotonergic dysfunction in BPD (2, 33). FEN is a serotonin-releasing agent, so a blunted response compared to placebo suggests reduced serotonergic activity. Both studies found an attenuated response to FEN in the orbital prefrontal region in patients relative to controls, supporting previous findings of dysfunction in this area. Siever et al. (2) reported a blunted response in the dorsolateral prefrontal region, though Soloff et al. did not. Siever et al. found a blunted response in the anterior cingulate region and right superior parietal lobe. Soloff et al. found a similar response in the left temporal lobe, left parietal lobe, and left caudate. Of note is that while Soloff et al. studied only patients with BPD (n=5), Siever et al. included patients (n=6) who had overlapping diagnoses of borderline, paranoid, and histrionic personality disorders.
Recent studies have used alpha-methyl-L-tryptophan (AMT) with PET to more directly evaluate serotonin synthesis capacity. AMT is converted to alpha-methyl-serotonin, which is not a substrate for monoamine oxidase and thus accumulates in the brain. This, in turn, may provide a more direct index of serotonin synthesis than previous techniques (35). Leyton et al. (1) studied AMT accumulation in patients with BPD (n=13) in conjunction with a test of behavioral disinhibition. They found that the patients made more errors than controls on a go/no-go task, and AMT accumulation inversely correlated with impulsivity scores. Affected regions included the orbital prefrontal region, anterior cingulate region (including the medial prefrontal gyrus), temporal cortex, and caudate (Figure 4). The authors concluded that low serotonin synthesis capacity in the corticostriatal pathways may contribute to impulsivity in BPD. While none of the patients had MDD or substance abuse at the time of evaluation, several had additional cluster B personality disorders and/or a history of mood disorders or substance abuse.
Conclusion
These studies are consistent in revealing dysfunction of interconnected prefrontal, limbic, and corticostriatal pathways that work together to process emotions and modulate behavior. They support the theory that reduced serotonin neurotransmission among these structures lowers the threshold for behavioral disinhibition, possibly involving downstream neurotransmitters such as gamma-aminobutyric acid or dopamine (2). They further suggest a multifaceted etiology that accounts not only for the impulsivity of BPD but also for affective lability, dissociation, and comorbid mood disorders.
While many of the individual findings from these studies do not appear to be unique to BPD, the combination may be, thereby producing its characteristic symptomatology. Differences in specific locations, boundaries, and laterality remain unclear but may relate to the few numbers of patients studied and myriad variations in approach and technique, making cross-comparisons challenging. Moreover, most of these studies have yet to be independently replicated and should therefore be considered exploratory. While not easily avoidable in BPD, many of these studies included patients with comorbid psychiatric disorders and substance abuse.
Despite these limitations, however, the findings are provocative and encouraging. They require further research to replicate these results and clarify implications. Neuroimaging promises to play an integral role in solving the mystery of emotion regulation in BPD, which in turn may guide future treatment strategies.
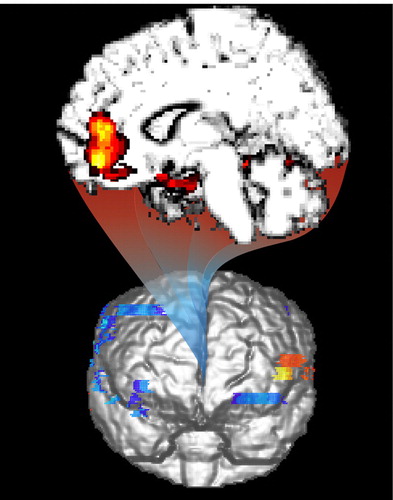
Figure 1. Magnetic resonance surface renderings of brain showing statistical probability mapping in patients with borderline personality disorder (BPD). (Top) PET scans demonstrate reduced accumulation of alpha-[11C ]methyl-L-tryptophan (AMT) in medial prefrontal, orbital prefrontal, and anterior cingulate cortices in patients relative to controls (reprinted with permission from Leyton et al. [1]). (Bottom) PET scans demonstrate reduced uptake of fluorodeoxyglucose (FDG) in response to d,l-fenfluramine (FEN) in similar regions of patients relative to controls (reprinted with permission from Siever et al. [2]).
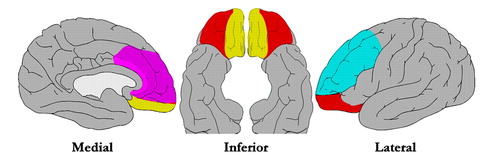
Figure 2. Regions of importance in emotion regulation. Anterior cingulate cortex (dark pink), adjacent medial prefrontal cortex (light pink), ventromedial prefrontal cortex (yellow), orbital prefrontal cortex (orange), and dorsolateral prefrontal cortex (blue) are illustrated on diagrams of the brain. These interconnected structures work together to generate, process, and control emotions. Pathology in any of these regions or in the pathways connecting them may result in dysregulation of emotions.
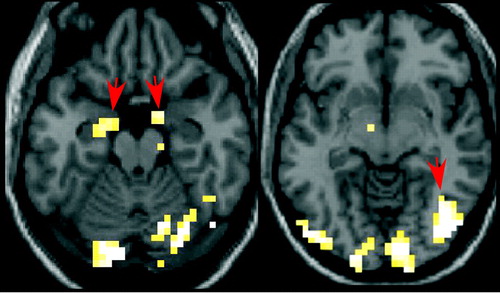
Figure 3. Functional magnetic resonance imaging (fMRI) regional cerebral hemodynamic changes in patients with BPD after viewing emotionally aversive slides. Subtracting the difference in changes after viewing aversive versus neutral slides in seven female patients from the difference in seven female controls revealed activation of the amygdala (arrows, left image) and fusiform gyrus (arrow, right image) in the patients, as indicated on axial magnetic resonance images (threshold=5.2, extent threshold=7, p<0.001 uncorrected).
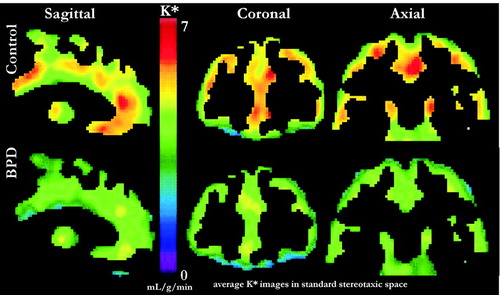
Figure 4. Positron emission tomography (PET) scans of a-[11C]methyl-l-tryptophan trapping in normal subjects (top row) compared to subjects with BPD (bottom row) indicate lower serotonin synthesis capacity in many regions, including medial, lateral, and orbital prefrontal cortex in BPD.
1 Leyton M, Okazawa, H, et al: Brain regional alpha-[11C]methyl-l-tryptophan trapping in impulsive subjects with borderline personality disorder. Am J Psychiatry 2001; 158:775–782Crossref, Google Scholar
2 Siever LJ, Buchsbaum MS, et al: d,l-fenfluramine response in impulsive personality disorder assessed with [18F]fluorodeoxyglucose positron emission tomography. Neuropsychopharmacology 1999; 20:413–423Crossref, Google Scholar
3 Skodol AE, Gunderson JG, et al: The borderline diagnosis I: psychopathology, comorbidity, and personality structure. Biol Psychiatry 2002; 51:936–950Crossref, Google Scholar
4 Skodol AE, Siever LJ, et al: The bordeline diagnosis II: biology, genetics, and clinical course. Biol Psychiatry 2002; 51:951–963Crossref, Google Scholar
5 Tyrer P: Practice guidelines for the treatment of borderline personality disorder: a bridge too far. J Personal Disord 2002; 16:113–118Crossref, Google Scholar
6 Torgersen S, Lygren S, et al: A twin study of personality disorders. Compr Psychiatry 2000; 41:416–425Crossref, Google Scholar
7 Zimmerman M, Mattia JI: Axis I diagnostic comorbidity and borderline personality disorder. Compr Psychiatry 1999; 40:245–252Crossref, Google Scholar
8 Skodol AE, Stout RL, et al: Co-occurrence of mood and personality disorders: a report from the collaborative longitudinal personality disorders study (CLPS). Depress Anxiety 1999; 10:175–182Crossref, Google Scholar
9 Linehan M: Cognitive-Behavioral Treatment of Borderline Personality Disorder. New York, NY, Guilford Press, 1993Google Scholar
10 Herpertz SC, Gretzer A, et al: Affective instability and impulsivity in personality disorder. Results of an experimental study. J Affective Disord 1997; 44:31–37Crossref, Google Scholar
11 Henry C, Mitropoulou V, et al: Affective instability and impulsivity in borderline personality and bipolar II disorders: similarities and differences. J Psychiatr Res 2001; 35:307–312Crossref, Google Scholar
12 Davidson RJ, Putnam KM, Larson CL: Dysfunction in the neural circuitry of emotion regulation: a possible prelude to violence. Science 7-28-2000; 289:591–594Crossref, Google Scholar
13 Royall DR, Lauterbach EC, et al: Executive control function: a review of its promise and challenges for clinical research. J Neuropsychiatry Clin Neurosci 2002; 14:377–405Crossref, Google Scholar
14 Battaglia M, Ferini-Strambi L, et al: Ambulatory polysomnography of never-depressed borderline subjects: A high-risk approach to rapid eye movement latency. Biol Psychiatry 1993; 33:326–334Crossref, Google Scholar
15 De la Fuente JM, Bobes J, et al: Sleep-EEG in borderline patients without comorbid depression: a comparison with major depressives and normal control subjects. Psychiatry Res 2001; 105:87–95Crossref, Google Scholar
16 Asaad T, Okasha T, Okasha A: Sleep EEG findings in ICD-10 borderline personality disorder in Egypt. J Affect Disord 2002; 71:11–18Crossref, Google Scholar
17 Benson KL, King R, et al: Sleep patterns in borderline personality disorder. J Affect Disord 1990; 18:267–273Crossref, Google Scholar
18 Battaglia M, Ferini-Strambi L, et al: First-cycle REM density in never-depressed subjects with borderline personality disorder. Biol Psychiatry 1999; 45:1056–1058Crossref, Google Scholar
19 Leibenluft E, Gardner DL, Cowdry RW: The Inner experience of the borderline self-mutilator. J Personal Disord 1987; 1:317–324Crossref, Google Scholar
20 Russ MJ, Campbell SS, et al: EEG theta activity and pain insensitivity in self-injurious borderline patients. Psychiatry Res 1999; 89:201–214Crossref, Google Scholar
21 Snyder S, Pitts, WM, Gustin Q: CT scans of patients with borderline personality disorder. Am J Psychiatry 1983; 140:272–272Google Scholar
22 Schulz SC, Koller MM, et al: Ventricular enlargement in teenage patients with schizophrenia spectrum disorder. Am J Psychiatry 1983; 140:1592–1595Crossref, Google Scholar
23 Lucas PB, Gardner DL, et al: Cerebral structure in borderline personality disorder. Psychiatry Res 1989; 27:111–115Crossref, Google Scholar
24 Lyoo IK, Han MH, Cho DY: A brain MRI study in subjects with borderline personality disorder. J Affect Disord 1998; 50:235–243Crossref, Google Scholar
25 Driessen M, Herrmann J, et al: Magnetic resonance imaging volumes of the hippocampus and the amygdala in women with borderline personality disorder and early traumatization. Arch Gen Psychiatry 2000; 57:1115–1122Crossref, Google Scholar
26 Bremner JD, Randall P, et al: Magnetic resonance imaging-based measurement of hippocampal volume in posttraumatic stress disorder related to childhood physical and sexual abuse: a preliminary report. Biol Psychiatry 1997; 41:23–32Crossref, Google Scholar
27 Bremner JD, Narayan M, et al: Hippocampal volume reduction in major depression. Am J Psychiatry 2000; 157:115–117Crossref, Google Scholar
28 Herpertz SC, Dietrich TM, et al: Evidence of abnormal amygdala functioning in borderline personality disorder: a functional MRI study. Biol Psychiatry 2001; 50:292–298Crossref, Google Scholar
29 Tebartz van Elst L, Thiel T, et al: Subtle prefrontal neuropathology in a pilot magnetic resonance spectroscopy study in patients with borderline personality disorder. J Neuropsychiatry Clin Neurosci 2001; 13:511–514Crossref, Google Scholar
30 Goyer PF, Andreason PJ, et al: Positron-emission tomography and personality disorders. Neuropsychopharmacology 1994; 10:21–28Crossref, Google Scholar
31 De la Fuente JM, Lotstra F, et al: Temporal glucose metabolism in borderline personality disorder. Psychiatry Res: Neuroimaging 1994; 55:237–245Crossref, Google Scholar
32 De la Fuente JM, Goldman S, et al: Brain glucose metabolism in borderline personality disorder. J Psychiatr Res 1997; 31:531–541Crossref, Google Scholar
33 Soloff PH, Meltzer CC, et al: A fenfluramine-activated FDG-PET study of borderline personality disorder. Biol Psychiatry 2000; 47:540–547Crossref, Google Scholar
34 Juengling FD, Schmahl C, et al: Positron emission tomography in female patients with borderline personality disorder. J Psychiat Res 2003; 37:109–115Crossref, Google Scholar
35 Young SN, Leyton M, Benkelfat C: PET studies of serotonin synthesis in the human brain in Tryptophan, Serotonin, and Melatonin: Basic Aspects and Applications. Edited by Huether, G, Kluwer Academic/Plenum Publishers, New York, 1999Google Scholar