Neuroimaging Studies of Psychological Interventions for Mood and Anxiety Disorders: Empirical and Methodological Review
Abstract
This article reviews the methods and results of published neuroimaging studies of the effects of structured psychological interventions for mood and anxiety disorders. The results are consistent with neural models of improved affective- and self-regulation, as evidenced by psychotherapeutic modulation of brain metabolic activity within the dorsolateral, ventrolateral, and medial prefrontal cortices, the anterior cingulate, the posterior cingulate/precuneus, and the insular cortices. Specific recommendations for future studies are outlined, and the clinical and theoretical significance of this research is discussed.
(Reprinted with permission from Clinical Psychology Review 28 (2008) 228–246)
Cognitive-, affective-, and social-psychology research are increasingly becoming integrated with the neuro-biological study of emotion, depression, and anxiety disorders under the banner of the social-cognitive and affective neurosciences (e.g., Davidson, 2000; Davidson et al., 2002; Lieberman, 2007; Murphy, Nimmo-Smith, & Lawrence, 2003; Ochsner & Gross, 2005). Indeed some researchers (e.g., Davidson et al., 2002) contend that the synthesis of psychological with neurobiological approaches to the study of depression and anxiety is of utmost importance to a comprehensive understanding of the etiology and effective treatment of these disorders.
Such interdisciplinary endeavors have been facilitated, in part, by the rapid development and research utilization of non-invasive methods for probing the neural processing underlying psychological functioning in humans, including in individuals with psychiatric disorders. These approaches include the functional neuroimaging technologies of positron emission tomography (PET) and functional magnetic resonance imaging (fMRI) (see Miller, Elbert, Sutton, & Heller, 2007, for review). PET and fMRI assessments measure tonic and/or discrete event-related metabolic activity as varying within distinct regions of the brain across time, such as indexed by glucose metabolic rate (PET) or blood oxygenation of vessels within surrounding neural tissue (fMRI); such metabolic activity is understood to indirectly assess neural activity as neural firing regionally impacts glucose and oxygen metabolism. Brain metabolic activity of individuals with and without psychiatric disorders can therefore be compared to identify the functional neural circuitry that may distinguish these groups. Pre-post changes in brain metabolic activity that occur with effective psychiatric treatment can also be examined to study the functional neural correlates of such treatment.
The present article reviews the experimental methodology and empirical results of 11 seminal neuroimaging studies published to date investigating the effects of participation in structured psychological interventions for mood and anxiety disorders on brain functioning. Specifically, the neural effects of cognitive-behavioural therapy (CBT) and interpersonal psychotherapy (IPT) are reviewed. Given that CBT and IPT are empirically-supported treatments for mood and anxiety disorders (Butler, Chapman, Forman, & Beck, 2006; Chambless & Ollendick, 2001; DeRubeis & Crits-Christoph, 1998; National Institute for Clinical Excellence, 2004), it may not be surprising that they might impact brain functioning in the direction of improved psychological health. These approaches are based on sound theoretical and empirically-tractable models of the psychological mechanisms involved in the etiology, maintenance, and amelioration of these disorders. Cognitive models posit that schemas or mental networks represent individual constellations of beliefs and attitudes about self, others, the environment, and experienced psychological and physiological symptoms. These schemas or mental networks are purported to moderate one's level of vulnerability to cognitive and affective disturbance within the context of stressful life events (Beck & Emery, 1985; Beck, Rush, Shaw, & Emery, 1979; Clark, Beck, & Alford, 1999; Ingram, Miranda, & Segal, 1998; Mathews & MacLeod, 2005). Although a multitude of CBT approaches have been tailored as interventions for specific mood and anxiety disorders, most therapies share the goals of regulating negative affect and arousal, restructuring negative self-referential cognition, improving problem-solving capacities, and increasing positive social-behavioural activity (Dobson & Dozois, 2001). Interpersonal models hypothesize that the way an individual perceives and is perceived by others within social relationships plays an integral role in vulnerability to mood and anxiety disorders (Coyne, 1976; Joiner & Coyne, 1999; Hammen, 1991; Segrin, & Dillard, 1992). Accordingly, interpersonal psychotherapy involves a concerted effort to restructure negative interpersonal relationships and foster positive social interactions as a means of decreasing negative affect and cognition related to depression and anxiety.
Studies conducted to date collectively suggest that CBT and IPT effectively alter brain function in individuals with unipolar mood disorders (Brody et al., 2001; Goldapple et al., 2004; Martin, Martin, Rai, Richardson, & Royall, 2001), obsessive–compulsive disorder (OCD; Baxter et al., 1992; Nakatani et al., 2003; Schwartz, Stoessel, Baxter, Martin, & Phelps, 1996), panic disorder (Praško et al., 2004), social anxiety disorder (Furmark et al., 2002), specific (spider) phobia (Paquette et al., 2003; Straube, Glauer, Dilger, Mentzel, & Miltner, 2006), and posttraumatic stress disorder (PTSD; Felmingham et al., 2007) in a manner that is consistent with the decreased psychiatric symptoms observed with these treatments. The present article reviews this emerging literature in detail to examine: (1) the clinical and theoretical significance of the empirical results for the study and treatment of mood and anxiety disorders, and (2) the validity of the research methods used in order to generate recommendations for future research.
The authors are aware of three previous articles that have been published that have also surveyed the neuroimaging of psychotherapy outcome literature (Beauregard, 2007; Linden, 2006; Roffman, Marci, Glick, Dougherty, & Rauch, 2005). However, neither of these reviews involved a detailed methodological evaluation nor was the focus specifically to distill the significance of this literature for clinical psychology theory and treatment of the mood and anxiety disorders. In contrast, the present article is motivated by the view that the introductory and formative status of the current literature affords an advantageous opportunity to determine a set of recommendations to guide future research of the effects of structured psychological interventions for mood and anxiety disorders on brain functioning. Additionally, this article reviews in detail the brain regions where activations and deactivations were observed in extant pre-post neuroimaging studies of CBT and IPT to ascertain the extent to which these results correspond with emerging data on the neurobiology of affective- and self-regulation (Ochsner & Gross, 2005; see also Beauregard, 2007).
NEUROIMAGING AND PSYCHOTHERAPY MECHANISMS OF CHANGE
Research on the mechanisms of change that relate to positive clinical outcomes in structured psychological interventions for mood and anxiety disorders is still very much in its infancy. A general assumption, however, is that clinical outcomes can be attributed to both common (e.g., the development of a positive therapeutic alliance) and specific factors (e.g., differential therapeutics). If the self-regulatory skills and strategies that are learned in interventions like CBT and IPT can be identified a priori, the psychological processes that hypothetically give rise to this learning can be investigated (e.g., DeRubeis et al., 1990; Ingram & Hollon, 1986), as can the neural correlates of these processes.
Generally speaking, CBT and IPT interventions involve at least three broad treatment goals (e.g., Dobson & Dozois, 2001). First, these interventions challenge clients' current ways of coping with stressful life events and problematic interpersonal relationships, and therefore attempt to enhance their problem-solving capacities. Studies of higher-order executive functioning processes such as working memory and cognitive flexibility generally suggest important roles for the dorsolateral prefrontal cortex (dlPFC; Cabeza & Nyberg, 2000; Duncan & Owen, 2000), making dlPFC functioning (and perhaps particularly how the dlPFC is deployed as a means of effectively resolving emotional-stressful situations), a possible target of CBT and IPT interventions. Second, CBT and IPT interventions attempt to modify clients' perspectives about themselves (self-representation) and their relationships. Neuroimaging studies suggest that cortical midline structures, including the ventral anterior cingulate cortex (vACC), dorsal anterior cingulate cortex (dACC), ventral and dorsal subregions of the medial prefrontal cortex (vmPFC, dmPFC), the posterior cingulate cortex, and the precuneus are collectively involved in self-related information processing (see Northoff et al., 2006 for review). Thus functioning within these brain areas are also potential therapeutic targets of CBT and IPT interventions. Third, CBT and IPT for mood and anxiety disorders attempt to assist individuals in regulating distressing affective states. Accordingly, these interventions may impact brain functions associated with emotional processing, such as within the insular cortex, the amygdala, the vACC and dACC, the dlPFC and ventrolateral PFC (vlPFC), and the vmPFC and dmPFC (see reviews by Murphy et al., 2003; Phan, Wager, Taylor, & Liberzon, 2003).
Finally, in addition to the results of therapeutic exercises specifically designed to increase problem-solving capacities, modify self-referential and relational processing, and improve affect regulation, clinical outcomes in CBT, IPT, and related interventions may also be dependent on, and partially attributable to, common psychotherapy factors, including the development of a positive therapeutic alliance. It is possible that the effects of the therapeutic alliance have their own distinctive set of neural substrates. However, another conceptualization is that factors associated with a strong therapeutic alliance, within structured psychological interventions, contribute directly or indirectly to adaptations taking place in problem-solving, self-referential and relational processing, and affect regulation (i.e., both common and specific factors may act on similar neural mechanisms).
Based on this review, it is apparent that an overlapping set of brain regions of interest is readily identifiable for studying the effects of structured psychological interventions for mood and anxiety disorders. These regions most prominently include the dlPFC, vlPFC, mPFC, ACC, PCC, amygdala, and insula. Given that the identification of these regions is based on psychological theory, investigations of the neural effects of CBT and IPT on mood and anxiety disorders may be conducted in a hypothesis-driven rather than purely exploratory manner. Accordingly, the empirical results of neuroimaging studies of CBT and IPT interventions for mood and anxiety disorders are reviewed below. The degree to which the results correspond with the hypothesis that these interventions impact the neural bases of affective- and self-regulatory processing is also considered (see also Beauregard, 2007).
EMPIRICAL REVIEW
IPT and CBT for major depression
Brody et al. (2001) and Martin et al. (2001) conducted the first neuroimaging studies of individuals with depression before and after a psychological intervention, both examining the effects of interpersonal psychotherapy (IPT). More recently Goldapple et al. (2004) investigated the neural effects of participation in cognitive therapy (CBT) for depression.
Brody et al. (2001) studied 14 unmedicated middle-aged adults recruited from their institution's surrounding community who received IPT in 12 weekly sessions (following Klerman, Weissman, Rounsaville, & Chevron, 1984). The IPT treated group was compared with a sample of paroxetine-treated depressed individuals (n=10) and with a non-psychiatric control group (n=16) of similar gender and age composition. The treatment arms of this study were assigned by participant preference rather than via randomized design. PET glucose metabolic rate was measured for all participants in an awake, restful state at baseline and again 12 weeks later. Regions of interest (ROIs) were defined as the dorsolateral and ventrolateral prefrontal cortex, dorsal and ventral anterior cingulate cortex, and dorsal and ventral caudate nucleus and thalamus. In the IPT group, pre-post decreases were observed in right prefrontal cortex, left anterior cingulate cortex, left insula, and left temporal cortex. Notably, however, differences in metabolism within the insula and temporal areas were not hypothesized a priori as ROIs, at least as explicitly reported in this article. Brody et al. also observed a significant correlation between change in left thalamic metabolism and change in HAM-D scores (τ=0.26). Although direct treatment comparisons of brain metabolic effects revealed mainly commonalities, metabolism increased bilaterally in the insula in the PT group whereas this occurred only within the left hemisphere in IPT participants.
Martin et al. (2001) investigated brain metabolic effects of IPT for depression as measured by single-photon emission computed tomography (SPECT). Twenty-eight middle-aged adults, who were referred by their family physicians and met DSM-IV criteria for Major Depressive Disorder (MDD) were studied. Twenty-three of these individuals were randomly assigned to receive either venlafaxine pharmacotherapy (PT, n=11) or IPT (n=12) (the remaining five individuals chose which treatment arm they would participate in [4 in PT; 1 in IPT]). Treatment outcome was determined by a single psychiatrist who was not blind to treatment arm and, in fact, administered the venlafaxine treatment. In comparison, all IPT sessions were conducted by a single psychiatric nurse, and patients received up to 16 one-hour weekly sessions. Participants were scanned prior to treatment and 6 weeks later, at which point treatment outcome was also determined. It is particularly noteworthy that the IPT participants were scanned only after their sixth therapy session. Thus the brain imaging results refer to mid-treatment as opposed to post-treatment outcome. Martin et al. observed increased blood flow in the right basal ganglia with both PT and IPT. Additionally, only participants treated with IPT showed increased blood flow in the right posterior cingulate over the course of treatment.
Finally, Goldapple et al. (2004) conducted the most recent neuroimaging investigation of pre- vs. post-psychological intervention in depressed individuals published to date. These investigators studied neural responses to cognitive therapy in 14 unmedicated depressed middle-aged outpatients who received CBT (15–20 sessions lasting a mean 26 weeks following Beck et al.'s (1979) cognitive therapy approach). The results of the CBT group were compared post-hoc with a previously-collected sample of paroxetine-treated (PT) depressed individuals (n=13) of similar depression severity at baseline (originally described in Kennedy et al., 2001). PET glucose metabolic rate was measured in the CBT patients within one-week prior to and after treatment in an awake and restful state, with no explicit instructions given except to avoid “ruminating on any one topic”(Goldapple et al., 2004, p. 35) during the PET fluid uptake phase. A similar scanning procedure was undertaken with the PT group prior to and following a six-week treatment interval, which is notably three-to-four times shorter than a standard CBT protocol. In the CBT group, Goldapple et al. found pre- vs. post-treatment increases in metabolic activity within the hippocampus/parahippocampal gyrus and dorsal cingulate cortex. Decreases were also found in dorsalateral and ventrolateral prefrontal regions, orbital frontal regions, the posterior cingulate, the inferior parietal regions, and in inferior temporal regions. Goldapple et al. also found that some of these changes were unique or in a different direction from the regional metabolic changes observed with PT in their previous study (Kennedy et al., 2001). Specifically, increased activation in the dorsal and decreased activation in the ventral subgenual cingulate, as well as decreased activation in the medial and ventrolateral prefrontal and orbital frontal regions were uniquely observed with CBT relative to PT. A converse pattern of activation to that observed in CBT was found with PT in the following areas: dorsolateral prefrontal, inferior parietal, and inferior temporal regions (where increases were found with PT), and hippocampus and parahippocampal regions (where decreases were found with PT).
As a set of initial findings, the results of these three studies suggest that participation in CBT and IPT for depression is associated with changes in brain metabolic activity in depressed individuals. Some consistent findings did emerge across the studies despite differences in the specific psychological interventions (IPT vs. CBT), neuroimaging methods, and follow-up periods utilized. Therapy outcomes were primarily associated with altered functioning in the prefrontal cortex (including both dorsolateral and ventrolateral, and medial regions) and in the cingulate cortex (including both anterior and posterior regions) during an awake state at rest. These findings are consistent with the idea that CBT and IPT help to alter psychological processing associated with problem-solving capacity, self-perception, and emotion, as hypothesized above. The dorsolateral prefrontal cortex, particularly within the left hemisphere, is widely known as a seat for many higher-order executive cognitive functions such as working memory and cognitive flexibility (Cabeza & Nyberg, 2000; Duncan & Owen, 2000). Altered function within the dorsolateral prefrontal cortex may reflect improved problem-solving (e.g., more effective coping with life stress) or may reflect reductions in negative affect and associated cognition such as involved in less worry and rumination. In contrast, altered activity within ventrolateral prefrontal regions, particularly within the right hemisphere, as well as within anterior and posterior cingulate and medial prefrontal regions may reflect improved affect regulation and self-perception, as these regions are thought to be involved in these psychological functions (Ochsner & Gross, 2005; Northoff et al., 2006).
CBT for OCD
Baxter et al. (1992) were the first to conduct a neuroimaging analysis of the effects of a structured psychological intervention on the brain, with their findings subsequently replicated and extended by the same research group (Schwartz et al., 1996). Their inaugural investigations probed the neural effects of CBT for OCD, and more recently a third study of the neural effects of CBT for OCD has also been published (Nakatani et al., 2003). Both Baxter et al. and Schwartz et al. investigated glucose metabolism using PET, whereas Nakatani et al. used xenon-enhanced computed tomography (Xe-CT) to study regional cerebral blood flow. Each of these studies investigated neural functioning in awake OCD patients during a restful state (i.e., participants were not involved in a specific psychological task while undergoing brain scanning). The studies consistently demonstrated altered functioning in the right caudate nucleus (part of the basal ganglia) with CBT, whereas Baxter et al. and Swartz et al. demonstrated altered functional connectivity of this region pre- versus post-CBT.
In a sample of 18 outpatients with OCD Baxter et al. (1992) predicted and confirmed a decrease in glucose metabolic rate in the right caudate nucleus in response to both pharmacotherapy (PT; n=9 [fluoxetine hydrochloride]) and CBT without medication (n=9). Participants were assessed before and after treatment, approximately 10 weeks later. The neuroimaging analyses were based upon twelve treatment responders (6 out of 9 in each condition). The percentage of change pre- vs. post-treatment in OCD severity (measured by the Yale-Brown Obsessive–Compulsive Scale [Y-BOCS]) was significantly associated with change in the metabolic rate of the right caudate with PT (Kendall's τ=0.48). A marginally significant correlation was also observed with CBT (τ=0.37). Baxter et al. also reported a differential pattern of correlations among these structures pre- vs. post-treatment that was consistent with alterations in a bilateral caudate nucleus–orbital frontal cortex–thalamic circuit occurring with successful treatment response. Specifically, correlated activity within these regions was significantly positive in treatment responders at baseline (τs ranged from 0.33 to 0.49) but was not significant at follow-up (τs −0.21 to 0.00). Although a positive correlation between left and right caudate glucose metabolic rate was not significant at baseline assessment (τ=0.28) it was so at follow-up (τ=0.77), with a similar pattern found for activity between the left caudate and left cingulate gyrus (pre-treatment, τ=−0.10, vs. post-treatment, τ=+0.41). No other significant differences were found in the direction or magnitude of correlations before versus after treatment. Although the caudate–orbital–thalamic circuit correlations were significantly altered within the full treatment-responsive sample, due to low sample sizes they were not evaluated separately for each treatment group, in the control group, or in treatment non-responsive participants. Given these selective analyses, coupled with the fact that treatment modality (CBT vs. PT) was participant elected rather than randomly assigned, the inferences that may be drawn about neural functioning related distinctly to CBT for OCD are limited in this study.
Accordingly Schwartz et al. (1996) replicated and extended these findings with an additional sample of nine unmedicated outpatients with OCD treated with CBT alone, six of whom were considered to be treatment responders and whose PET data were submitted to further analysis. The finding from the Baxter et al. (1992) study of a significant decrease in the metabolic rate of the right caudate nucleus with CBT-response was replicated. Moreover, combining the results from both the CBT-treated samples in both studies, Schwartz et al. also demonstrated a significant decrease in the metabolic rate of the left caudate. No additional baseline vs. follow-up differences were observed. Schwartz et al. also replicated the finding of a marginally significant positive correlation between percentage change pre- vs. post-treatment in the glucose metabolic rate of the right (τ=0.32) and left (τ=0.26) caudate with percentage change in Y-BOCS scores. A similar pattern of pre- vs. post-differential correlations between structures in the caudate–orbital–thalamic circuit was identified when the Baxter et al. and Schwartz et al. CBT treatment-responding samples were combined (correlations before treatment ranged from 0.34 to 0.81, and between −0.01 and +0.41 after treatment).
A more recent neuroimaging investigation of CBT for OCD was completed by Nakatani et al. (2003), who also studied awake resting-state brain function in 22 Japanese individuals with OCD. Thirty-one individuals with OCD were initiated into the treatment trial, although eight did not improve and were not scanned at the conclusion of the study (another participant's data were not interpretable due to excessive head movement during scanning). In contrast to the standardized treatment duration implemented in the two previous investigations (approximately 10 weeks; Baxter et al., 1992; Schwartz et al., 1996), CBT was continued in the Nakatani et al. study until a given participant was considered, by consensus of the clinical and researcher team, to be responsive to treatment. The method used to determine treatment response was not, however, detailed explicitly. Notably the mean duration between pre- and post-treatment testing in Natakani et al.'s study was 7.55 months, on average more than twice as long as in Baxter et al. and Schwartz et al.; treatment duration was also significantly heterogenous across participants (SD=4.68). A noteworthy limitation of this study was that all but one of the participants who completed the study had been maintained on Clomipramine throughout the duration of the study, making uncertain the extent to which the brain alterations observed over the course of treatment could be attributed to CBT, PT, or their interaction. Minding these methodological limitations, Nakatani et al. also demonstrated a reduction in blood flow within the right head of the caudate nucleus over the course of the CBT intervention. Furthermore, increases in functional status (Global Assessment of Functioning Scale scores) were significantly correlated with decreases in right caudate metabolism (r=−0.46).
As a set of preliminary results, the findings from these three studies are intriguing and consistent with what is currently known about the neurobiology of OCD from resting-state neuroimaging studies (reviewed by Friedlander & Desrocher, 2006). Collectively these studies suggest that response to exposure and response-prevention programs, as determined by a reduction in OCD symptoms, is associated with a significant right hemisphere or bilateral reduction in the metabolic rate of the caudate, and that the percentage of change in the metabolic rate of the caudate correlates with clinical change. Additionally, Baxter et al. (1992) and Schwartz et al. (1996) demonstrated that alteration in the metabolic activity of a caudate–orbital–thalamic brain circuit was associated with a positive treatment response to CBT. The caudate nuclei, part of the basal ganglia, are known to be involved in the execution of motor-behaviour as partly coordinated by the motor, premotor, and dorsolateral prefrontal cortices, and this system has been implicated in the executive dysfunction and compulsive behaviour accompanying OCD symptomatology (Friedlander & Desrocher, 2006). Therefore, these findings may reflect enhanced self-regulatory control in CBT-treated individuals with OCD. Specifically, these findings may signify decreased motor output in the form of reduced compulsive behaviour, or improved inhibition of compulsive behaviour.
CBT for panic disorder
Praško et al. (2004) are the first and only published investigators to examine the effects of CBT for panic disorder as assessed by a neuroimaging design. These researchers studied six non-medicated individuals with non-comorbid panic disorder before and after a twelve-week group CBT intervention (the proportion of participants who also met diagnostic criteria for agoraphobia was not reported). CBT consisted of three 1.5 h sessions conducted weekly for the first 6 weeks, and two-additional booster sessions were administered during the eighth and twelfth week. The CBT protocol consisted of psychoeducation, cognitive restructuring, instruction in diaphragmatic breathing and relaxation, and interoceptive and in vivo exposure to panic symptom provocation. Participants were randomly assigned to the CBT treatment, with a comparison group of six individuals randomized to receive antidepressant treatment (citalopram [n=3], sertraline [n=2], or venlafaxine [n=1]). Between group (CBT vs PT) comparisons were not, however, investigated in this study.
Glucose metabolic rate was examined over 30 min in an awake at-rest state (as in the investigations reviewed above) using PET. Praško et al. (2004) observed increased metabolism at post-treatment within an array of brain regions that included the left inferior and right middle frontal cortex, left insula, right precuneus, and right posterior cingulate cortex, as well as in the left middle and superior temporal cortex and middle and superior parietal cortex.
As this is the first neuroimaging study of a psychological treatment for panic disorder, these findings require replication before their functional significance can be properly elucidated. Indeed, the direction of pre-post differences observed in this study was inconsistent with the majority of other neuroimaging of psychotherapy outcome studies. The findings, however, appear to be consistent with the proposed mechanisms of change in CBT for panic disorder (e.g., altering problem-solving capacity and emotional processing). For instance, it is possible to speculate that increased metabolism within the left insula would be partly reflective of an increase in parasympathetic (relative to sympathetic) tone (Craig, 2005), indicative of individuals' increased ability to ‘feel at ease’ within their physical body and surrounding environment as a result of the CBT intervention. Similarly, increased metabolism within medial posterior regions (right precuneus and posterior cingulate) may be implicative of a greater preponderance of ‘resting-state’ brain activity and reflective function (Gusnard & Raichle, 2001), that is, a more passive or decentered information-processing mode, akin to a brain less actively engaged in or excessively motivated by the need to monitor potentially threatening interoceptive (e.g., heart-rate) and exteroceptive (e.g., escape route) stimuli.
CBT for social anxiety disorder
Furmark et al. (2002) conducted the only thus far published neuroimaging investigation of the effects of CBT for social phobia. Eighteen previously untreated middle-aged adults who met diagnostic criteria for social phobia, without comorbid diagnoses, were studied. Participants were randomized to Cognitive Behavioural Group Therapy (based on Hope & Heimberg, 1993), Citalopram pharmacotherapy (PT), or a wait-list control condition. These groups were matched for the number of individuals who had been diagnosed with generalized (versus non-generalized) social phobia (three per group). Oxygen 15-labeled water PET scans were acquired for all participants pre- and post-treatment (12 weeks later) during an anxiety-provoking public speaking task. Specifically, 20 min before scanning, participants were asked to prepare a short speech about a vacation or travel experience and, while being scanned, performed this speech in the presence of at least a six-person audience while being recorded by a portable video-camera. State anxiety before and after this task was measured via self-report on a 100-point Subjective Units of Distress scale as well as with the Spielberger State-Anxiety Inventory. Both treatment groups evidenced significant reductions on psychometric indices of social phobia. The wait-list control group, in contrast, showed no clinically appreciable change on any of the measures. The PT and CBT groups did not differ significantly on any outcome measure post-treatment and both of these groups reported less state anxiety during the speech task post-relative to pre-treatment.
In the CBT group, reduced blood flow was observed bilaterally in the amygdala, hippocampus, periaqueductal gray, and anterior and medial temporal cortex, while increases in blood flow in the right cerebellum and the secondary visual cortex were observed. In contrast, no significant changes in cerebral blood flow were observed in these areas within the wait-list control group. The only significant difference that was observed in a between-group comparison of changes associated with CBT versus PT was an increase in blood flow in the right thalamus that was observed with PT only.
Since these findings are based on only a single study, future replication is necessary before their clinical significance can be determined with any degree of certainty. However, the diminished public speaking fears participants experienced with treatment would be consistent with reduced activity in the amygdala. Additionally, reduced activity within the hippocampus and medial temporal lobe may relate to a perceived lower need to consolidate the post-treatment public speaking experience as a threatening situation to be avoided in the future, as these areas have been implicated in fear conditioning and emotional episodic memory (e.g., review by Phelps, 2006).
Strengths of the Furmark et al. (2002) study included the use of a functional task-paradigm with clear relevance to the anxiety disorder under investigation (i.e., public speaking), and the inclusion of a wait-list control group with randomization to treatment, although small sample sizes were employed. Additionally, a strength of this study involved the use of self-report and physiological (heart-rate) monitoring of anxiety during the experimental paradigm as a means of insuring that the neural responses studied pre- versus post-treatment were relevant to clinical psychological change. However, associations between these indices and brain responses were not examined directly in an individual differences design.
CBT for spider phobia
Paquette et al. (2003) and Straube et al. (2006) examined the neural correlates of successful cognitive-behavioural treatment of spider phobia using fMRI. In each case, participants viewed images of spiders while blood-oxygenation-level dependent (BOLD) neural responses were recorded before and after CBT.
Paquette et al. (2003) studied twelve medication-free early-adult-aged females with non-comorbid spider phobia before and after a four-session group CBT program for spider phobia. Prior to being enrolled in the study, potential participants viewed film excerpts of spiders in a simulated MRI environment and were selected for participation if these films evoked in them an experience of intense but tolerable fear, as measured by self-report. Thirteen female non-psychiatric controls were also recruited for comparison purposes at baseline, each of whom were selected based on their absence of fear-responsiveness to the same spider film excerpts. Whereas individuals with spider phobia were assessed both before and after treatment, control participants completed the scanning task on only a single occasion for pre-treatment comparison purposes.
CBT was delivered in the Paquette et al. (2003) study in three-hour intensive group sessions (6 participants per group) conducted once per week for four consecutive weeks. Treatment followed a graded exposure model. This intervention incorporated homework exercises involving viewing still pictures and films of spiders and, by the final session, all participants successfully touched a live tarantula without self-reported fear.
Before and after the CBT exposure regimen, participants in the Paquette et al. (2003) study completed a functional neuroimaging task. In this task, participants viewed ten blocks of 30 s film excerpts which alternated between depictions of living spiders in captivity (the experimental task) and butterflies occupied in nature (the control or reference task). The order of excerpts was counterbalanced across participants. Immediately after the scanning session, participants rated, on a 9-point scale (0= ‘absence of fear’; 8=‘fear as intense as if real spiders were touching a phobic subject's body’), the degree to which they experienced fear while viewing the spider excerpts. Spider phobic participants reported experiencing considerable fear during this spider film-viewing task (M=6.3, SD=1.2) whereas the non-psychiatric control group reported limited (if any) experience of fear in response to the same paradigm (M=0.4, SD=0.7). It was noteworthy that post-scan debriefing after the pre-treatment scanning session revealed that a large number of the individuals with spider phobia had attempted to control their fear during the task by voluntarily engaging controlled breathing. Attesting to a successful treatment response, the spider phobia group reported a near absence of fear post-CBT (M=0.1, SD=0.3) with their post-treatment self-report fear ratings now statistically indistinguishable from those of the control participants.
After isolating BOLD responses in the brain uniquely associated with viewing spider film excerpts, individuals with spider phobia displayed significantly greater activation than did controls in the dorsolateral prefrontal cortex and parahippocampul gyrus before treatment. Within this group, significant activations were also observed in the right inferior frontal gyrus and the occipital cortex (left inferior-occipital gyrus, left fusiform gyrus, and right middle occipital gyrus). Following CBT, significant reductions in BOLD activity of the dorsolateral prefrontal cortex and parahippocampul gyrus were found in the spider phobia group, whereas the right inferior frontal gyrus was more significantly active post-treatment than pre-treatment. This increased activity within the right inferior frontal gyrus was attributed to improved emotion regulation processes within the context of exposure to a previously feared stimulus (Ochsner & Gross, 2005).
The Paquette et al. study demonstrated several methodological strengths, including the use of a neuroimaging paradigm with clear relevance to the psychiatric disorder under investigation that proved sensitive to treatment effects in terms of self-reported response. However, there were also weaknesses of this study, perhaps most noteworthy of which was that the film excerpts used in the neuroimaging scans were the same as those used in CBT sessions. This fact raises the possibility that the neural changes observed pre- to post-treatment may have been the consequence of repeated testing rather than exposure therapy per se. This methodology is especially problematic given that the neural responses of control participants were only examined at baseline rather than repeatedly and that a spider phobia wait-list control group was not included in the study (Straube et al., 2006).
Straube et al. (2006) remedied some of these methodological problems. These investigators compared 28 women with spider phobia to 14 women without psychiatric diagnosis. Twenty-five of the 28 women with spider phobia participated in a second scanning session held 2 weeks later, 13 of whom had been randomized to receive CBT for their spider phobia in the interim, and 12 of whom had been randomized to a wait-list control group (the remaining three participants were lost to follow-up due to missed scans [n=2] or an aborted scan due to the occurrence of panic attack [n=1, the latter was a wait-list control participant]). CBT was conducted over two four-to-five hour sessions (following procedures outlined by Öst, 1989, 1996) that were held on two successive days that began the day following pre-treatment scanning. Their treatment involved psychoeducation in addition to an exposure protocol that included training toward holding a living tarantula for approximately 10 min, in addition to catching and touching other live spiders without excessive self-reported fear (as measured by a score of less than 50 on a 100-point subjective units of distress scale). Apparently, all participants reached these therapy goals Straube et al. (2006).
The task used by Straube et al. (2006) during scanning involved viewing 24 s videos depicting either a moving spider (experimental task) or, during separate epochs, a moving black cylinder (control task). None of these images were used as stimuli within the treatment itself. As in the Paquette et al. (2003) study, participants rated, on an interval scale, the extent to which they experienced fear in response to this experimental paradigm. Whereas the spider phobia group as a whole predictably rated the experimental spider videos as more fear-inducing than did the non-psychiatric control participants, the CBT-treated spider phobic participants evidenced a reduction in such ratings following the intervention, whereas the wait-list group did not show an appreciable reduction in their fear ratings over the same time interval.
At baseline, spider phobia participants evidenced greater BOLD activation bilaterally in the anterior insular and anterior cingulate cortices, as well as in the left occipital cortex (lingual gyrus) than non-psychiatric controls. In contrast, non-psychiatric controls showed increased BOLD activation within the left amygdala, bilaterally in the parahippocampus gyrus, and within the pre- and post-central gyri. No significant between-group differences between the CBT and wait-list spider phobia groups were observed at baseline. Whereas the CBT group no longer demonstrated a greater response in the insular and anterior cingulate cortex to viewing spiders relative to the cylindrical control object at the follow-up assessment, the wait-list group continued to reveal these effects, and these differences significantly distinguished the groups in a direct between-groups contrast. Additionally, at the second assessment, the wait-list group exhibited greater response in the thalamus, left dorsomedial prefrontal cortex, and left precuneus, whereas the CBT group revealed greater response in the right precuneus. Moreover, the post-treatment results did not differ between the CBT and non-psychiatric control groups. In contrast, neural responses to the spider videos continued to differentiate the wait-list group (second scanning session) from the non-psychiatric controls (baseline scanning). Specifically, the spider phobia group who had not received treatment continued to exhibit significantly greater response within the anterior insula and anterior cingulate cortex. The findings of lowered anterior cingulate and right insular cortex responses to the previously feared spider stimuli in individuals treated with CBT are consistent with lowered negative affective responses, as both structures are strongly implicated in negative emotional responses that generate autonomic nervous system activity (Phan, Wager et al., 2003; Murphy et al., 2003).
Together the results of Paquette et al. (2003) and Straube et al. (2006) reveal significant differences in brain activation to phobic objects following cognitive-behavioural treatment, which elucidate some of the neural underpinnings of the altered affective experience of these objects as reported by the participants in their studies. Specifically, their findings appear to be consistent with improved affect regulation and coping within the context of exposure to a previously feared stimulus. It is surprising, however, that a limited overlap in the neural regions identified was observed between the two studies given the similar scanning methods. Further studies are therefore necessary to determine which regions most reliably mediate the CBT effects of treatment of spider phobia as well as other specific phobias. The consistently admitted use of controlled breathing by the spider phobia group studied by Paquette et al. during pre-treatment may raise interpretative problems. Specifically, this finding, although presumably clinically reflective of the fear and anxious arousal provoked in response to the phobic stimuli (and perhaps unavoidable as such), is a behaviour that differentiated this groups' responses to the paradigm at baseline from those evoked within the non-psychiatric control group with which they were compared. This fact may therefore account for the differences in brain response between the groups, specifically, that although both groups were instructed only to attend toward the films during scanning, the spider phobic individuals additionally attended toward and attempted to regulate their breathing during scanning. This attempted self-regulatory act may therefore partially account for the between-group differences at baseline and the within-group pre-post differences at follow-up. The frequency of occurrence of this behaviour was not noted explicitly in the study by Straube et al. In general, however, the findings are consistent with CBT altering psychological processing associated with emotional processing function.
CBT for PTSD
The most recent contribution to the neuroimaging of psychological treatment outcome literature has been made by Felmingham et al. (2007). These researchers studied the results of an exposure-based cognitive-behavioural intervention for PTSD in a pre–post design (a control group was not available for comparison). Five women and three men who had developed PTSD following an assault (n=4) or a car accident (n=4) were studied at an average of 65 months (SD=64) following their traumatic event. Four of these individuals met diagnostic criteria for comorbid major depression, and two individuals were taking selective serotonin reuptake inhibitors over the period in which the study testing took place. The CBT for PTSD intervention encompassed imaginal exposure to participants' traumatic memories coupled with cognitive restructuring over eight sessions (see Bryant, Moulds, Guthrie, Dang, & Nixon, 2003). Such treatment was associated with a reduction in PTSD symptoms as measured by the Clinician-Administered PTSD Scale, upon which participants obtained a mean score of 78.1 at pre-treatment and 28.9 at post-treatment (6 months later).
Before and 6 months following treatment, participants viewed pictures of fearful and neutral faces while undergoing fMRI scanning of their brains. The dependent measure of interest was unique responses to fearful relative to neutral facial expressions, and a priori brain regions of interest to this comparison were defined as the ventral anterior cingulate cortex and the amygdala. Other brain regions were also examined using a more stringent significance threshold.
Felmingham et al. determined that ventral ACC was activated more significantly post-relative to pre-treatment bilaterally, whereas amygdala activation was not observed at either time point. Felmingham et al. also observed that changes in PTSD symptom severity correlated positively with change in right ACC activation (r=0.84), and negatively with change in right amygdala activation (r=−0.85). In their exploratory analysis, Felmingham et al. also demonstrated that activation within the right post-central gyrus, right middle temporal gyrus, and left superior temporal gyrus was greater before than after treatment, whereas the reverse was found within the left middle temporal gyrus, right inferior frontal gyrus, left parietotemporal gyrus, and right hippocampus. The authors' concluded that the pattern of findings for the ACC and amygdala are consistent with improved fear extinction, although that their study requires replication with larger samples and inclusion of a wait-list control condition.
Summary
As a set of introductory findings, the 11 studies reviewed above collectively offer preliminary support for the notion that participation in structured psychological interventions for mood and anxiety disorders, such as CBT and IPT, is associated with alterations in neural functioning, specifically changes in brain blood flow and oxygen/glucose metabolism. More specifically, the empirical results suggest that CBT and IPT interventions impact neural functioning in a manner that is consistent with the amelioration of mood and anxiety disorder symptoms, and the results are also consistent with emerging neural models of affective- and self-regulation (e.g., Davidson, 2000; Lieberman, 2007; Ochsner & Gross, 2005). The cumulative results of these studies that were observed at cortical levels (excluding occipital cortex) are illustrated in Fig. 1.
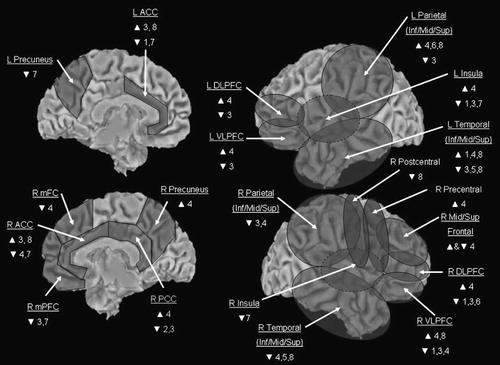
Note: Circles illustrate general location. The more medial location of the insula is indicated by the hatched lines. Data for occipital cortex and non-cortical regions (e.g., thalamus, basal ganglia) are not illustrated. 1 = Brody et al. (2001), 2 = Martin et al. (2001), 3 = Goldapple et al. (2004), 4 = Praško et al. (2004), 5 = Furmark et al. (2002), 6 = Paquette et al. (2003), 7 = Straube et al. (2006), 8 = Felmingham et al. (2007), L = left, R = right, ACC = anterior cingulate cortex, DLPFC = dorsolateral prefrontal cortex, VLPFC = ventrolateral prefrontal cortex, Inf/Mid/Sup = inferior/middle/superior, mFC = medial frontal cortex, mPFC = medial prefrontal cortex, PCC = posterior cingulate cortex.
Thus, broadly speaking, the findings are collectively consistent with the notion that CBT and IPT interventions for mood and anxiety disorders alter brain functioning associated with problem-solving, self-referential and relational processing, and affect regulation. For example, both anterior and posterior cortical midline structures that are related to self-referential processing (Northoff et al., 2006) were found to be impacted by CBT and IPT interventions, as were sites known to be involved in the regulation of negative affect, such as regions within ventral and dorsal ACC, mPFC, and right ventrolateral (inferior frontal) cortex (Ochsner & Gross, 2005). Studies have also found that activity within the left dlPFC was modulated by psychotherapy for mood and anxiety disorders, which may be consistent with increased executive functioning (e.g., improvements in working memory, and/or increased and/or more effective deployment of working memory in problem-solving solutions to difficult emotional and stressful situations; Zelazo & Cunningham, 2007).
Clearly, however, future research is needed to better elucidate the functional and clinical significance of these findings. Therefore, methodological recommendations for use in future neuroimaging studies of the effects of structured psychological interventions are outlined below, in order to increase the theoretical and clinical merit that might be derived from future studies.
METHODOLOGICAL REVIEW
The studies reviewed above, to a varying degree, exhibit procedural strengths that are pertinent to all forms of treatment outcome research, such as the incorporation of formal diagnostic methods, psychometric testing, and behavioural ratings that indicate the degree of symptom severity pre- versus post-treatment. However, additional studies will be required before firm conclusions can be drawn about potential direct causal links between participation in structured psychotherapeutic interventions, such as CBT and IPT, and changes in brain function reflective of improved psychological health. For example, a limitation common to all extant studies involves the relatively small sample size used, which limits the generalizability of the findings. Accordingly, future studies are advised to incorporate larger samples. Additional recommendations for future neuroimaging studies of psycho-therapy outcome are offered below, with the intention of maximizing the validity of future study designs, and capitalizing on the degree to which future studies will be able to definitively address important questions about the neural effects of CBT, IPT, and other structured psychological interventions for mood and anxiety disorders. These recommendations are described in detail below, and additionally outlined in brief in Table 1 for easy reference. Table 1 also evaluates each of the studies reviewed in this article according to the degree to which it exhibits the recommended characteristics of a methodologically sound neuroimaging study of psychotherapy outcome as advocated herein.1
![]() |
Table 1. Recommendations for Future Neuroimaging Research of the Effects of Psychological Interventions on Brain Functioning
Recommendations
The first three recommendations listed in Table 1 are relatively straightforward. The first general recommendation is to include non-psychiatric control groups at pre-treatment for baseline comparison purposes. Only half of the studies reviewed above included a non-psychiatric control group for comparison purposes at pre-treatment (Baxter et al., 1992; Brody et al., 2001; Nakatani et al., 2003; Paquette et al., 2003; Straube et al., 2006). These exclusions make interpretation of the findings difficult by prohibiting unambiguous determination of the psychological significance of the distinguishing patterns of brain function demonstrated by clinical participants at baseline. As Thase (2001) astutely noted, if abnormal brain functioning is not confirmed for clinical participants at baseline (i.e., in comparison with non-psychiatric controls), it precludes sensibility to expect participants' possibly already conceivably ‘normal’ brain function at baseline to ‘normalize’ further with treatment. Equally important, it is prudent for researchers to assess the representativeness of the neuroimaging profiles of their clinical samples at baseline (i.e., relative to previously conducted neuroimaging studies that have compared the same clinical groups to non-psychiatric controls). Non-replication of previously published group differences at baseline may suggest that the sample under investigation is unique, whereas replication would imply that the neuroimaging outcomes occurring with psychotherapy in a given study may be generalizable to the population of individuals with the same psychiatric disorder being investigated.
Second, it is advisable for studies to include a wait-list control group with the psychiatric disorder under investigation, and that an absence of differences in neuroimaging results between the treated and wait-list groups is established at baseline. Only the Furmark et al. (2002) and Straube et al. (2006) studies included wait-list control groups, and only the latter explicitly reported whether their treatment and wait-list groups differed with respect to baseline neuroimaging profiles. The inclusion of wait-list control groups diagnosed with the psychiatric disorder being investigated is essential as a methodological control for brain changes that may occur merely in association with repeated testing and/or over time.
For example, particularly in cases where participants have not been acclimatized to the scanning environment itself prior to baseline testing, subsequent scanning sessions may be perceived as more or less stressful than a previous session(s) as a consequence of experiences taking place during the earlier sessions. Moreover, it is possible that state-functional neural images of contrasts between groups of individuals with psychiatric disorders in comparison with controls may unduly reflect state anxiety-arousal engendered by the scanning procedure itself. In other words, although fears about the imaging scanner and scanning environment may be germane to the symptoms that are definitive of various specific psychiatric disorders (e.g., fear of being unable to escape from within the bore of an MRI scanner in an individual suffering from agoraphobia), research questions of interest typically do not directly concern the neural correlates of anxiety reactions associated specifically with brain scanning. Thus the question of whether neural differences between groups might be confounded by state anxiety engendered by the scanning procedure warrants judicious consideration. It therefore seems advisable that participants' level of fear concerning the scanning procedure itself be measured, and potentially used as a regressor of interest on the brain results obtained. This is arguably especially the case for resting-state studies where clinical participants with anxiety disorders may find themselves in a decidedly less ‘restful’ state than control participants during brain scanning. Briefly, a third recommendation, one critical for any study seeking to establish causal significance, is that participants be randomized to the various treatment conditions under study. In less than half of the studies reviewed above were participants randomized to treatment conditions (see Table 1).
A fourth methodological recommendation is that, whenever possible, investigators should conduct both resting-state and functional neuroimaging analyses at the pre- and post-treatment intervals. Furthermore, it will be important for future studies to examine possible associations between resting-state and functional brain responses in individuals with and without the psychiatric disorders under investigation. None of the previously published studies included both resting-state and functional-experimental neuroimaging conditions.
The inclusion of both resting-state and functional response paradigms is important because the two neuroimaging designs are likely to index different neural facets of clinical psychological presentation. Resting-state studies are important to examine as they may be particularly indicative of the tonic clinical presentation of individuals, and they are conceivably less susceptible than are functional paradigms to experimental demand effects (and by nature practice effects). Additionally, there has been increasing interest in the cognitive neuroscience community in understanding the neural processing underlying the resting or ‘default mode’ state in humans (e.g., Fox, Corbetta, Snyder, Vincent, & Raichle, 2006; Fransson, 2005, 2006; Grecius, Krasnow, Reiss, & Menon, 2003). Indeed certain investigators envision that this literature may bear significantly on our ultimate understanding of cognition-emotion interactions and of psychiatric symptomatology (e.g., Drevets & Raichle, 1998). It is possible that resting-state findings may be most indicative of a general marker of psychopathological disturbance. For example, by the DSM-IV criteria, a symptom bearing on the diagnosis of many forms of affective disorder is that of the individual's perceived difficulty with maintaining his or her concentration and attention, and disturbances in memory also often accompany the diagnosis of mood and anxiety disorders. Differences in resting-state neural function, for example, may be particularly sensitive to these types of general cognitive markers of psychopathology. In studies of this nature, however, it is important that investigators ensure that during the resting-state period psychiatric and non-psychiatric study participants are similarly engaged. For example, psychological processing such as degree of self- versus external-focused attention, frequency of intrusive thought or rumination, and degree of state anxiety or arousal experienced during the resting-state period may differ systematically across individuals with the psychiatric disorder under investigation. Again, in this situation, the experimental conditions may not be parallel in the clinical and control participants, perhaps then posing interpretative barriers on valid inferences about brain activity in the two groups, particularly when such behaviour is not effectively measured (i.e., by self-report). Such measurements may also be pertinent to the valid interpretation of resting blocks interspersed within functional paradigms as well, particularly in cases where resting blocks are frequent and/or lengthy. Given that neural activity occurring during active time points within a functional neuroimaging paradigm is often ascertained as a comparison with neural activity occurring during resting blocks, it is important to verify that clinical and control participants are similarly engaged during the resting portions of the paradigm.
Although resting-state studies are important to conduct, several theorists have proposed that vulnerability to psychopathology is most appropriately studied when participants' dispositions toward experiencing abnormal psychological states are in a ‘primed’ or active state (e.g., Ingram et al., 1998). For example, theorists have proposed that vulnerability to depression is conferred primarily when negative self-referential processing is activated during periods of sad-dysphoric mood; current cognitive theories hold that depression-vulnerable individuals may not otherwise appear different from non-psychiatric controls during more euthymic or less affectively-toned states (Ingram et al., 1998). Thus functional paradigms that prime disorder-relevant psychological-neural processing may be more sensitive to group differences between individuals with mood and anxiety disorders in comparison with controls and, accordingly, neuroimaging paradigms that invoke such processing may be more sensitive to group and pre-post treatment differences as well. Additionally, to the degree that functional tasks hold constant the information processing taking place during scanning within (if not between) clinical and control groups, the neural activations that are observed may be more easily interpretable than those obtained from resting-state studies. Specifically, the unconstrained nature of the psychological processing that participants may be involved in during resting-state studies can serve to delimit the number of plausible interpretations that might ultimately be made about the results that are obtained at a psychological level of analysis.
At the same time, fashioning an experimental paradigm that comprehensively probes all of the psychological processes clinically relevant to a given psychiatric disorder is probably not possible. In other words, the psychological tasks used in neuroimaging studies are likely to measure only certain facets of a disorder, while being less sensitive to other components of an overall clinical presentation. An illustration is available from the posttraumatic stress disorder (PTSD) literature, where a paradigm commonly employed is trauma script-driven imagery, in which participants are scanned while listening to audio-scripts that recount their traumatic experiences. This paradigm consistently provokes re-experiencing symptoms (e.g., sensory flashbacks, intense emotion, and physiological hyperarousal) and/or dissociation in individuals with PTSD, and the neural correlates of these experiences have been studied (reviewed by Lanius, Bluhm, Lanius, & Pain, 2006). However, whereas this paradigm may effectively index psychological processing that is involved in the re-experiencing and dissociative symptoms of PTSD, it may not be a particularly good indicator of the psychological processing underlying these individuals' emotional numbing symptoms, which nevertheless are arguably just as integral to complex clinical presentations of PTSD (Frewen & Lanius, 2006).
A fifth recommendation listed in Table 1 is that researchers collect self-report, other behavioural, and/or other psychophysiological data during or immediately after the neuroimaging scanning. As examples, such data might represent Likert-scale emotion ratings in response to an affect generation paradigm, response times in a vigilance-detection task, or heart-rate or skin-conductance level in response to an arousal manipulation. Not only can such data validate that an expected experimental manipulation took place (i.e., a clinical group responded more strongly to the manipulation than did a control group, or vice versa), but between-participant variability in these data can also be incorporated as regressors of activity in brain regions of interest, as appropriate to the research questions of interest. The majority of the functional neuroimaging studies of psychotherapy outcome conducted to date (Furmark et al., 2002; Paquette et al., 2003; Straube et al., 2006) collected self-report ratings, although none of these studies tested these ratings as predictors of brain activity in their statistical analytic designs (see Table 1). Specifically, collection of these data respects potential individual differences that may emerge not only between clinical and non-clinical groups but also within each group in terms of neuropsychological response to an experimental paradigm. Therefore, whereas a clinical group as a whole may show differences from a control group, within the clinical group there may still remain a significant level of variability in responsiveness to a particular neuroimaging paradigm. Incorporation of this individual variability in one's modeling of neural responses may account for a greater amount of variance in the neuroimaging signal measured in comparison with a statistical model that solely attributes variability in neural processing to group-level or task-indexed differences (e.g., Hopper, Frewen, van der Kolk, & Lanius, in press; Phan, Taylor et al., 2003; Phan, Wager et al., 2003).
A sixth recommendation is that neuroimaging data be correlated with symptom severity, including analysis of both treatment responders and non-responders. Several of the previously conducted studies tested correlations between brain responses and psychometric scores that measured psychiatric symptom severity. However, several studies also excluded treatment non-responders from the outcome analysis. In contrast, where symptom change is measured continuously as opposed to dichotomously, such variation in outcome can be correlated with variation in brain response, an analytic strategy that may more feasibly be inclusive of all pre-treatment participants in the post-treatment analysis, a desirable quality on both methodological as well as statistical grounds. It may also be important to measure the degree to which participants' experience psychiatric symptoms during scanning (e.g., level of depressed mood in a depressed participant) rather than only retrospectively (e.g., average level of depressed mood in depressed individual over the previous week/month). Measuring state symptoms in addition to measuring averaged symptoms over a longer duration is important because the effect of the latter may be partly moderated by the degree of the former.
In addition to symptom-based measures, a final recommendation is that investigators incorporate psychometric measures of change on variables theoretically purported to mediate symptom reduction in a given psychotherapeutic intervention. This recommendation would theoretically afford investigators the opportunity to examine the neural correlates of change associated with the mediating psychological variable, in addition to brain changes signifying symptom remission, which may or may not significantly overlap. In other words, while analyses of symptom change alone may primarily reveal overlapping neural correlates of the effects of psychological and pharmacological interventions, as found in several studies reviewed here (Baxter et al., 1992; Brody et al., 2001; Furmark et al., 2002; Martin et al., 2001), analyses of psychological change may reveal brain changes that are distinctively the result of structured psychological interventions. For example, within CBT interventions for depression, the intention of clinicians is typically to teach participants, among other things, self-monitoring skills (e.g., awareness of negative automatic thoughts) and skills in cognitive reappraisal as means of regulating affective states and altering self-schemas. However, whereas at post-treatment a majority of individuals may be classified non-depressed based on self-report (i.e., survey or clinical interview), participants may nevertheless vary considerably with respect to how effectively they learned (a) the self-monitoring and cognitive reappraisal skills, and (b) the degree to which such learning mediated the depressive symptom reduction they experienced across time (e.g., in comparison with participants treated to symptom remission via pharmacotherapy). If such variation in learning and its mediation of symptom change can be systematically quantified, independently of variation in depressive symptoms (e.g. with a validated psychometric scale, interview, or experimental response measure), pre-post treatment change scores on such a measure can be correlated with pre-post change in brain responses. This kind of analysis would facilitate the identification of brain regions that may be directly associated with and perhaps mediate the increased coping and self-regulatory skills learned in a treatment like CBT, which may indeed not be identical to the neural correlates of symptom remission. In investigations of this nature, future studies may choose to follow the statistical analytic methodology for testing mediation outlined by Baron and Kenny (1986).
Finally, previous studies have exclusively focused on describing the neural correlates of CBT and IPT relative to either PT or no-treatment. In contrast, no published neuroimaging studies have compared the effects of different structured psychological interventions, or even compared the effects of structured psychological interventions like CBT and IPT to less structured psychological interventions (e.g., supportive therapy). Thus, for studies seeking to identify the neural correlates of specific therapeutic factors, psychotherapy control groups will need to be included. A common research strategy along these lines is to compare the efficacy of a structured psychological intervention to a less structured one as a means of controlling for non-specific aspects of psychotherapy (e.g., positive therapeutic alliance). Rather than including forms of psychotherapy previously shown to be sub-optimal as treatment for a particular mood or anxiety disorder, however, an alternative means of assessing the role of the therapeutic alliance is as a moderator or mediator of treatment outcome within an already empirically-supported structured psychological intervention. In this way, the possible direct and indirect neural effects of participation in a healing relationship, such as that engendered by certain forms of psychotherapy, can be probed using neuroimaging designs without requiring inclusion of a ‘placebo-based’ psychological treatment. Examination of the effects of the therapeutic alliance may be a particularly productive research strategy in studies of the effects of psychotherapy for psychiatric disorders that are, arguably, inherently relational (e.g., atypical depression, social anxiety disorder, posttraumatic stress disorder associated with interpersonal violence and/or abuse). Furthermore, studies in social-cognitive neuroscience are beginning to uncover how perceived level of social support modulates neural responses to anxiety (e.g., threat of shock; Coan, Schaefer, & Davidson, 2006) and social exclusion (Eisenberger, Taylor, Gable, Hilmert, & Lieberman, 2007). These methods may be profitably adapted to future studies of the strength of the therapeutic relationship as a moderator or mediator of the neural changes taking place over effective psychological treatments for mood and anxiety disorders.
In summary, the studies reviewed above lay the groundwork for additional methodologically rigorous and theoretically sophisticated tests of the effects of psychological interventions on brain function to be conducted in the future. As such, the present juncture is an opportune time to identify standards for the conduct of future investigations of the neural effects of structured psychological interventions for mood and anxiety disorders. Table 1 lists the above provisional recommendations for future studies seeking to test the effects of psychotherapeutic interventions on brain functioning in different psychiatric disorders (see also Beutel, Stern, & Silbersweig, 2003, for a set of general recommendations regarding research collaborations conducted between neuroscientists and psychotherapists).
CONCLUSION
Although a general movement toward acceptance of the biopsychosocial model of psychiatric disorders largely characterizes the current zeitgeist in mental health research, for some an underlying viewpoint is that each of the three dimensions of the bio-psycho-social model contributes relatively independently to the development of psychiatric disorders. For example, a premorbid neurobiological risk factor (e.g., genetic liability) might be viewed as a predisposing factor for individuals to experience psychiatric problems under specific conditions of elevated psychosocial risk or stress. This kind of an explanation of the biopsychosocial model resounds with an underlying conceptualization of the biological, psychological, and sociological factors contributing relatively independently (either additively or interactively) to the development of the mental and behavioural phenomena that may ultimately be diagnosed as a psychiatric disorder.
An alternative viewpoint is that psychosocial mechanisms are reflected in neurobiology, just as pathological disturbances in neurobiological systems may express themselves causally in psychosocial behaviour. This point of view examines each of the tri-perspectives of the bio-psycho-social model at different levels of analysis rather than as independent contributing factors. This perspective is therefore consistent with the idea that psychosocial experiences manifest or express themselves in the brain by altering its structure and function, one general framework that has been put forward for understanding the effects of psychotherapy on the brain (Cozolino, 2002). The view does not, however, posit that either neurobiological or psychosocial factors are more or less ‘important’ than each other, or that one definitively causes the other. Instead, the model is fully consistent with a bi-directional influence occurring between brain, behaviour, and environment that can be studied at each of these levels.
Which distinctive conceptualization of the biopsychosocial model one adopts is a decision of considerable consequence, because it may partially determine one's approach to treatment. For example, espousal of the separatist-interactive view would be consistent with the notion that the biological and psychological components of psychiatric disorders may require separate treatments, the biological component perhaps treated by pharmacotherapy and the psychological component via a structured psychological intervention like CBT or IPT (e.g., Elkin, Pilkonis, Docherty, & Sotsky, 1988). This dualistic conception therefore promotes the use of combination psychosocial and medical modes to treatment. In contrast, an integrative or ‘levels-of-analysis’ perspective would posit that psychological interventions also directly impact brain functioning, just as pharmacological interventions directly impact psychological functioning. This perspective may lead less to combination psychological and pharmacological interventions, or to more optimal use of combination treatments (e.g., a pharmacological agent known to impact a particular type of learning is used to maximize learning and change associated with a psychological intervention, as in recent work of the effects of d-Cycloserine as a moderator of the effects of exposure-based treatments for anxiety disorders; Hofmann et al., 2006; Ressler et al., 2004; see Davis, Ressler, Rothbaum, & Richardson, 2006 for review).
The neuroimaging evidence reviewed above, that in individuals with mood and anxiety disorders structured psychological interventions such as CBT and IPT alter brain metabolism in neural sites that are consistent with these therapies' effects appears inconsistent with the separatist-interactionist view. Collectively, the evidence reviewed above is consistent with the hypothesis that participation in structured psychological interventions is associated with observable changes in brain functioning over time. Moreover, the functional brain changes that have been observed to accompany participation in structured psychological interventions for mood and anxiety disorders, broadly construed, appear to be consistent with improved affective self-regulation (see Fig. 1). However, as also reviewed above, several limitations of this emerging literature are readily identifiable, and future studies to extend these early investigations may benefit by incorporating some of the methodological suggestions listed in Table 1.
Given that the theoretical synthesis of psychological with neurobiological perspectives has been regarded by some as the most important endeavor for advancing a unified science and treatment of psychiatric disorders (e.g., Andreasen, 1997; Davidson et al., 2002; Kendall, 1998), it is expected that many more investigations of the effects of psychotherapy on the brain will be conducted in the near future. Hopefully these studies will move us toward a better understanding of the suffering experienced by individuals with mood and anxiety disorders, and how participation in structured psychological interventions helps alleviate this suffering.
Andreasen, N. C. (1997). Linking mind and brain in the study of mental illnesses: A project for a scientific psychopathology. Science, 275, 1586–1593. Crossref, Google Scholar
Baron, R. M., & Kenny, D. A. (1986). The moderator-mediator variable distinction in social psychological research: Conceptual, strategic, and statistical considerations. Journal of Personality and Social Psychology, 51, 1173–1182. Crossref, Google Scholar
Baxter, L. R., Schwartz, J. M., Bergman, K. S., Szuba, M. P., Guze, B. H., Mazziotta, J. C., et al. (1992). Caudate glucose metabolic rate changes with both drug and behavior therapy for obsessive–compulsive disorder. Archives of General Psychiatry, 49, 681–689. Crossref, Google Scholar
Beauregard, M. (2007). Mind does really matter: Evidence from neuroimaging studies of emotional self-regulation, psychotherapy, and placebo effect. Progress in Neurobiology, 81, 218–236. Crossref, Google Scholar
Beck, A. T., & Emery, G. (1985). Anxiety disorders and phobias: A cognitive perspective. New York: Basic Books. Google Scholar
Beck, A. T., Rush, A. J., Shaw, B. F., & Emery (1979). Cognitive therapy of depression. New York, NY: Guilford Press. Google Scholar
Beutel, M. E., Stern, E., & Silbersweig, D. A. (2003). The emerging dialogue between psychoanalysis and neuroscience: Neuroimaging perspectives. Journal of the American Psychoanalytic Association, 51, 773–801. Crossref, Google Scholar
Brody, A. L., Saxena, S., Stoessel, P., Gillies, L. A., Fairbanks, L. A., Alborzian, S., et al. (2001). Regional brain metabolic changes in patients with major depression treated with either paroxetine or interpersonal therapy: Preliminary findings. Archives of General Psychiatry, 58, 631–640. Crossref, Google Scholar
Bryant, R. A., Moulds, M. L., Guthrie, R. M., Dang, S. T., & Nixon, R. D. V. (2003). Imaginal exposure alone and imaginal exposure with cognitive restructuring in treatment of posttraumatic stress disorder. Journal of Consulting and Clinical Psychology, 71, 706–712. Crossref, Google Scholar
Butler, A. C., Chapman, J. E., Forman, E. M., & Beck, A. T. (2006). The empirical status of cognitive-behavioral therapy: A review of meta-analyses. Clinical Psychology Review, 26, 17–31. Crossref, Google Scholar
Cabeza, R., & Nyberg, L. (2000). Imaging cognition II: An empirical review of 275 PET and fMRI studies. Journal of Cognitive Neuroscience, 12, 1–47. Crossref, Google Scholar
Chambless, D. L., & Ollendick, T. H. (2001). Empirically supported psychological interventions: Controversies and evidence. Annual Review of Psychology, 52, 685–716. Crossref, Google Scholar
Clark, D. A., Beck, A. T., & Alford, B. A. (1999). Scientific foundations of cognitive theory and therapy of depression. New York, NY: Wiley. Google Scholar
Coan, J. A., Schaefer, H. S., & Davidson, R. J. (2006). Lending a hand: Social regulation of the neural response to threat. Psychological Science, 17, 1032–1039. Crossref, Google Scholar
Coyne, J. C. (1976). Toward an interactional description of depression. Psychiatry, 39, 28–40. Crossref, Google Scholar
Cozolino, L. J. (2002). The neuroscience of psychotherapy: Building and rebuilding the human brain. New York, NY: W W Norton & Co. Google Scholar
Craig, A. D. (2005). Forebrain emotional asymmetry: A neuroanatomical basis? Trends in Cognitive Sciences, 9, 566–571. Crossref, Google Scholar
Davidson, R. J. (2000). Emotion, plasticity, context, and regulation: Perspectives from affective neuroscience. Psychological Bulletin, 126, 890–909. Crossref, Google Scholar
Davidson, R. J., Lewis, D. A., Alloy, L. B., Amaral, D. G., Bush, G., Cohen, J. D., et al. (2002). Neural and behavioural substrates of mood and mood regulation. Biological Psychiatry, 52, 478–502. Crossref, Google Scholar
Davis, M., Ressler, K., Rothbaum, B. O., & Richardson, R. (2006). Effects of d-Cycloserine on extinction: Translation from preclinical to clinical work. Biological Psychiatry, 60, 369–375. Crossref, Google Scholar
DeRubeis, R. J., & Crits-Christoph, P. (1998). Empirically supported individual and group psychological treatments for adult mental disorders. Journal of Consulting and Clinical Psychology, 66, 37–52. Crossref, Google Scholar
DeRubeis, R. J., Evans, M. D., Hollon, S. D., Garvey, M. J., Grove, W. M., & Tuason, V. B. (1990). How does cognitive therapy work? Cognitive change and symptom change in cognitive therapy and pharmacotherapy for depression. Journal of Consulting and Clinical Psychology, 58, 862–869. Crossref, Google Scholar
Dobson, K. S., & Dozois, D. J. A. (2001). Historical and philosophical bases of the cognitive-behavioral therapies. In K. S. Dobson (Ed.), Handbook of cognitive-behavioral therapies (pp. 3–39). New York, NY: Guilford Press. Google Scholar
Drevets, W. C., & Raichle, M. E. (1998). Suppression of regional cerebral blood flow during emotional versus higher cognitive processes: Implications for interactions between emotion and cognition. Cognition and Emotion, 12, 353–385. Crossref, Google Scholar
Duncan, J., & Owen, A. M. (2000). Common regions of the human frontal lobe recruited by diverse cognitive demands. Trends in Neurosciences, 23, 475–483. Crossref, Google Scholar
Eisenberger, N. I., Taylor, S. E., Gable, S. L., Hilmert, C. J., & Lieberman, M. D. (2007). Neural pathways link social support to attenuated neuroendocrine stress responses. Neuroimage, 35, 1601–1612. Crossref, Google Scholar
Elkin, I., Pilkonis, P. A., Docherty, J. P., & Sotsky, S. M. (1988). Conceptual and methodological issues in comparative studies of psychotherapy and pharmacotherapy: I. Active ingredients and mechanisms of change. American Journal of Psychiatry, 145, 909–917. Crossref, Google Scholar
Felmingham, K., Kemp, A., Williams, L., Das, P., Hughes, G., Peduto, A., et al. (2007). Changes in anterior cingulate and amygdala after cognitive behavior therapy of posttraumatic stress disorder. Psychological Science, 18, 127–129. Crossref, Google Scholar
Fox, M. D., Corbetta, M., Snyder, A. B., Vincent, J. L., & Raichle, M. E. (2006). Spontaneous neuronal activity distinguishes human dorsal and ventral systems.
Fransson, P. (2005). Spontaneous low-frequency BOLD signal fluctuations: An fMRI investigation of the resting-state default mode of brain function hypothesis. Human Brain Mapping, 26, 15–29. Crossref, Google Scholar
Fransson, P. (2006). How default is the default mode of brain function?: Further evidence from intrinsic BOLD signal fluctuations. Neuropsychologia, 44, 2836–2845. Crossref, Google Scholar
Frewen, P. A., & Lanius, R. A. (2006). Toward a psychobiology of posttraumatic self-dysregulation: Re-experiencing, hyperarousal, dissociation, and emotional numbing. Annals of the New York Academy of Sciences, 1071, 110–124. Crossref, Google Scholar
Friedlander, L., & Desrocher, M. (2006). Neuroimaging studies of obsessive–compulsive disorder in adults and children. Clinical Psychology Review, 26, 32–49. Crossref, Google Scholar
Furmark, T., Tillfors, M., Marteinsdottir, I., Fischer, H., Pissiota, A., Langstrom, B., et al. (2002). Common changes in cerebral blood flow in patients with social phobia treated with citalopram or cognitive-behavioral therapy. Archives of General Psychiatry, 59, 425–433. Crossref, Google Scholar
Goldapple, K., Segal, Z., Garson, C., Lau, M., Bieling, P., Kennedy, S., et al. (2004). Modulation of cortical-limbic pathways in major depression: Treatment-specific effects of cognitive behavior therapy. Archives of General Psychiatry, 61, 34–41. Crossref, Google Scholar
Grecius, M. D., Krasnow, B., Reiss, A. L., & Menon, V. (2003). Functional connectivity in the resting brain: A network analysis of the default mode hypothesis.
Gusnard, D. A., & Raichle, M. E. (2001). Searching for a baseline: Functional imaging and the resting human brain. Nature Reviews Neuroscience, 2, 685–694. Crossref, Google Scholar
Hammen, C. (1991). Generation of stress in the course of unipolar depression. Journal of Abnormal Psychology, 100, 555–561. Crossref, Google Scholar
Hofmann, S. G., Meuret, A. E., Smits, J. A., Simon, N. M., Pollack, M. H., Eisenmenger, K., et al. (2006). Augmentation of exposure therapy with d-cycloserine for social anxiety disorder. Archives of General Psychiatry, 63, 298–304. Crossref, Google Scholar
Hope, D. A., & Heimberg, R. G. (1993). Social phobia and social anxiety. In D. H. Barlow (Ed.), Clinical handbook of psychological disorders: A step-by-step treatment manual (pp. 99–136). New York, NY: Guilford Press. Google Scholar
Hopper, J. W., Frewen, P. A., van der Kolk, B. A., & Lanius, R. A. (in press). Dimensional analysis of responses to script-driven trauma imagery in PTSD: Neural correlates of re-experiencing, avoidance, and dissociation. Journal of Traumatic Stress. Google Scholar
Ingram, R. E., & Hollon, S. D. (1986). Cognitive therapy for depression from an information processing perspective. In R. E. Ingram (Ed.), Information processing approaches to clinical psychology (pp. 259–281). San Diego, CA: Academic Press. Google Scholar
Ingram, R. E., Miranda, J., & Segal, Z. V. (1998). Cognitive vulnerability to depression. New York, NY: Guilford Press. Google Scholar
Joiner, T., & Coyne, J. C. (Eds.). (1999). The interactional nature of depression: Advances in interpersonal approaches. Washington: American Psychological Association. Google Scholar
Kendall, P. C. (1998). Empirically supported psychological therapies. Journal of Consulting and Clinical Psychology, 66, 3–6. Crossref, Google Scholar
Kennedy, S. H., Evans, K. R., Kruger, S., Mayberg, H. S., Meyer, J. H., McCann, S., et al. (2001). Changes in regional brain glucose metabolism measured with positron emission tomography after paroxetine treatment of major depression. American Journal of Psychiatry, 158, 899–905. Crossref, Google Scholar
Klerman, G. L., Weissman, M. M., Rounsaville, B. J., & Chevron, E. S. (1984). Interpersonal Psychotherapy of Depression. New York, NY: Basic Books. Google Scholar
Lanius, R. A., Bluhm, R., Lanius, U., & Pain, C. (2006). A review of neuroimaging studies in PTSD: Heterogeneity of response to symptom provocation. Journal of Psychiatric Research, 40, 709–729. Crossref, Google Scholar
Lieberman, M. D. (2007). Social cognitive neuroscience: A review of core processes. Annual Review of Psychology, 58, 259–289. Crossref, Google Scholar
Linden, D. E. J. (2006). How psychotherapy changes the brain: The contribution of functional neuroimaging. Molecular Psychiatry, 11, 528–538. Crossref, Google Scholar
Martin, S. D., Martin, E., Rai, S. S., Richardson, M. A., & Royall, R. (2001). Brain blood flow changes in depressed patients treated with interpersonal psychotherapy or venlafaxine hydrochoride: Preliminary findings. Archives of General Psychiatry, 58, 641–648. Crossref, Google Scholar
Mathews, A., & MacLeod, C. (2005). Cognitive vulnerability to emotional disorders. Annual Review of Clinical Psychology, 1, 167–195. Crossref, Google Scholar
Miller, G. A., Elbert, T., Sutton, B. P., & Heller, W. (2007). Innovative clinical assessment technologies: Challenges and opportunities in neuroimaging. Psychological Assessment, 19, 58–73. Crossref, Google Scholar
Murphy, F. C., Nimmo-Smith, I., & Lawrence, A. D. (2003). Functional neuroanatomy of emotions: A meta-analysis. Cognitive, Affective, & Behavioral Neuroscience, 3, 207–233. Crossref, Google Scholar
Nakatani, E., Nakgawa, A., Ohara, Y., Goto, S., Uozumi, N., Iwakiri, M., et al. (2003). Effects of behavior therapy on regional cerebral blood flow in obsessive-compulsive disorder. Psychiatry Research: Neuroimaging, 124, 113–120. Crossref, Google Scholar
National Institute for Clinical Excellence (2004). Anxiety: Management of anxiety (panic disorder, with or without agoraphobia, and generalized anxiety disorder) in adults in primary, secondary and community care. Retrieved from www.nice.org.uk/CG022quickrefguide on January 17, 2007. Google Scholar
Northoff, G., Heinzel, A., de Greck, M., Bermpohl, F., Dobrowolny, H., & Panksepp, J. (2006). Self-referential processing in our brain: A meta-analysis of imaging studies of the self. Neuroimage, 31, 440–457. Crossref, Google Scholar
Ochsner, K. N., & Gross, J. G. (2005). The cognitive control of emotion. Trends in Cognitive Sciences, 9, 242–249. Crossref, Google Scholar
Öst, L. -G. (1989). One-session treatment for specific phobias. Behaviour Research & Therapy, 27, 1–7. Crossref, Google Scholar
Öst, L. -G. (1996). One-session group treatment of spider phobia. Behaviour Research & Therapy, 34, 707–715. Crossref, Google Scholar
Paquette, V., Lévesque, J., Mensour, B., Leroux, J. -M., Beaudoin, G., Bourgouin, P., et al. (2003). “Change the mind and you change the brain: Effects of cognitive-behavioral therapy on the neural correlates of spider phobia. Neuroimage, 18, 401–409. Crossref, Google Scholar
Phan, K. L., Taylor, S. F., Welsh, R. C., Decker, L. R., Noll, D. C., Nichols, T. E., et al. (2003). Activation of the medial prefrontal cortex and extended amygdala by individual ratings of emotional arousal: A fMRI study. Biological Psychiatry, 53, 211–215. Crossref, Google Scholar
Phan, K. L., Wager, T., Taylor, S. F., & Liberzon, I. (2003). Functional neuroanatomy of emotion: A meta-analysis of emotion activation studies in PET and fMRI. Neuroimage, 16, 331–348. Crossref, Google Scholar
Phelps, E. A. (2006). Emotion and cognition: Insights from studies of the human amygdala. Annual Review of Psychology, 57, 27–53. Crossref, Google Scholar
Praško, J., Horácek, J., Zálesky, R., Kopecek, M., Novák, T., Pašková, B., et al. (2004). The change of regional brain metabolism (18FDG PET) in panic disorder during the treatment with cognitive behavioral therapy or antidepressants. Neuroendocrinology Letters, 5, 340–348. Google Scholar
Ressler, K. J., Rothbaum, B. O., Tannenbaum, L., Anderson, P., Graap, K., Zimand, E., et al. (2004). Cognitive enhancers as adjuncts to psychotherapy: Use of D-Cycloserine in phobic individuals to facilitate extinction of fear. Archives of General Psychiatry, 61, 1136–1144. Crossref, Google Scholar
Roffman, J. L., Marci, C. D., Glick, D. M., Dougherty, D. D., & Rauch, S. L. (2005). Neuroimaging and the functional neuroanatomy of psychotherapy. Psychological Medicine, 35, 1385–1398. Crossref, Google Scholar
Schwartz, J. M., Stoessel, P. W., Baxter, L. R., Martin, K. M., & Phelps, M. E. (1996). Systematic changes in cerebral glucose metabolic rate after successful behavior modification treatment of obsessive-compulsive disorder. Archives of General Psychiatry, 53, 109–113. Crossref, Google Scholar
Segrin, C., & Dillard, J. P. (1992). The interactional theory of depression: A meta-analysis of the research literature. Journal of Social and Clinical Psychology, 11, 43–70. Crossref, Google Scholar
Straube, T., Glauer, M., Dilger, S., Mentzel, H. -J., & Miltner, W. H. R. (2006). Effects of cognitive-behavioral therapy on brain activation in specific phobia. Neuroimage, 29, 125–135. Crossref, Google Scholar
Thase, M. E. (2001). Neuroimaging profiles and the differential therapies of depression. Archives of General Psychiatry, 58, 651–653. Crossref, Google Scholar
Zelazo, P. H., & Cunningham, W. A. (2007). Executive function: Mechanisms underlying emotion regulation. In J. G. Gross (Ed.), Handbook of emotion regulation (pp. 135–158). New York: Guildford Press. Google Scholar