Atypical Antipsychotics: Mechanism of Action
Abstract
Background: Although the principal brain target that all antipsychotic drugs attach to is the dopamine D2 receptor, traditional or typical antipsychotics, by attaching to it, induce extrapyramidal signs and symptoms (EPS). They also, by binding to the D2 receptor, elevate serum prolactin. Atypical antipsychotics given in dosages within the clinically effective range do not bring about these adverse clinical effects. To understand how these drugs work, it is important to examine the atypical antipsychotics’ mechanism of action and how it differs from that of the more typical drugs. Method: This review analyzes the affinities, the occupancies, and the dissociation time-course of various antipsychotics at dopamine D2 receptors and at serotonin (5-HT) receptors, both in the test tube and in live patients. Results: Of the 31 antipsychotics examined, the older traditional antipsychotics such as trifluperazine, pimozide, chlorpromazine, fluphenazine, haloperidol, and flupenthixol bind more tightly than dopamine itself to the dopamine D2 receptor, with dissociation constants that are lower than that for dopamine. The newer, atypical antipsychotics such as quetiapine, remoxipride, clozapine, olanzapine, sertindole, ziprasidone, and amisulpride all bind more loosely than dopamine to the dopamine D2 receptor and have dissociation constants higher than that for dopamine. These tight and loose binding data agree with the rates of antipsychotic dissociation from the human-cloned D2 receptor. For instance, radioactive haloperidol, chlorpromazine, and raclopride all dissociate very slowly over a 30-minute time span, while radioactive quetiapine, clozapine, remoxipride, and amisulpride dissociate rapidly, in less than 60 seconds. These data also match clinical brain-imaging findings that show haloperidol remaining constantly bound to D2 in humans undergoing 2 positron emission tomography (PET) scans 24 hours apart. Conversely, the occupation of D2 by clozapine or quetiapine has mostly disappeared after 24 hours. Conclusion: Atypicals clinically help patients by transiently occupying D2 receptors and then rapidly dissociating to allow normal dopamine neurotransmission. This keeps prolactin levels normal, spares cognition, and obviates EPS. One theory of atypicality is that the newer drugs block 5-HT2A receptors at the same time as they block dopamine receptors and that, somehow, this serotonin-dopamine balance confers atypicality. This, however, is not borne out by the results. While 5-HT2A receptors are readily blocked at low dosages of most atypical antipsychotic drugs (with the important exceptions of remoxipride and amisulpride, neither of which is available for use in Canada) the dosages at which this happens are below those needed to alleviate psychosis. In fact, the antipsychotic threshold occupancy of D2 for antipsychotic action remains at about 65% for both typical and atypical antipsychotic drugs, regardless of whether 5-HT2A receptors are blocked or not. At the same time, the antipsychotic threshold occupancy of D2 for eliciting EPS remains at about 80% for both typical and atypical antipsychotics, regardless of the occupancy of 5-HT2A receptors. Relevance: The “fast-off-D2” theory, on the other hand, predicts which antipsychotic compounds will or will not produce EPS and hyperprolactinemia and which compounds present a relatively low risk for tardive dyskinesia. This theory also explains why l-dopa psychosis responds to low atypical antipsychotic dosages, and it suggests various individualized treatment strategies.
Definition of typical and atypical antipsychotic drugs
Traditional or “typical” antipsychotics such as haloperidol and chlorpromazine, when used in clinically effective dosages, induce elevated levels of serum prolactin, extrapyramidal signs and symptoms (EPS) and, after a period of time, tardive dyskinesia (TD). However, antipsychotic drugs such as olanzapine, clozapine, quetiapine, and amisulpride are “atypical” because, in contrast to the traditional antipsychotics, they elicit low or negligible levels of these untoward side effects while still effectively controlling psychotic symptoms.
Which neuron pathway is clinically most affected by antipsychotic drugs?
Immediately after the clinical introduction of drugs for psychosis (1), clinicians observed that patients taking these medications exhibited a Parkinson-like syndrome of tremor, akinesia, and rigidity (2). This drug-induced parkinsonism strongly suggested that antipsychotic drugs were interfering with dopamine pathways in the human brain, because Parkinson’s disease was known to be a disease of insufficient dopamine neurotransmission. This clinical observation gave birth to the dopamine hypothesis of psychosis and antipsychotic drug action (3).
Although it was suggested that chlorpromazine and haloperidol blocked “5-hydroxytryptamine (serotonin) and monoaminergic (noradrenaline and dopamine) receptors” (4), it was not possible at that time to conclude which of the 3 pathways was selectively affected by antipsychotics. This is because the turnover of noradrenaline, serotonin (5-HT), and dopamine were all simultaneously affected by the antipsychotics (4, 5). Andén and others speculated that chlorpromazine and haloperidol “reduce the elimination rates of these” metabolites of noradrenaline, 5-HT, and dopamine (5). Although Andén and others (6) subsequently found that antipsychotic drugs in vivo had a greater effect on dopamine turnover than on noradrenaline turnover, direct in vitro evidence for the selective blockade of dopamine receptors was found only later (7–9).
The multiple clinical and adverse effects of various antipsychotic drugs depend on the combination of receptors occupied, but the dopamine pathway is the primary common target for all antipsychotic drugs. More specifically, “no drug has yet been identified with antipsychotic action without a significant affinity for the D2 receptor” (10, 11).
Which group of dopamine receptors is clinically relevant for antipsychotic drug action?
There are 5 types of dopamine receptors in human beings (12, 13). Types 1 and 5 are similar in structure and drug sensitivity (14, 15), and these 2 receptors are referred to as the “D1-like” group or class of receptors. Dopamine receptor types 2, 3, and 4 are also similar in structure and are, therefore, grouped together as the “D2-like” group. Dopamine receptors 2, 3 and 4, however, have significantly different sensitivities to antipsychotic drugs.
Al though the D1-like receptors are often mentioned as a primary target for antipsychotic drugs (16), 3 findings indicate that the D1-like receptors are not clinically relevant in the therapeutic action of these drugs. First, D1 antagonists do not clinically improve psychotic signs and symptoms (17–19). Second, therapeutic maintenance dosages of various antipsychotic drugs occupy low or negligible levels of D1 receptors in the brains of patients with psychosis (20). For example, therapeutic dosages of haloperidol occupy less than 5% of the dopamine receptors in the brain putamen of schizophrenia patients (20). Although therapeutic dosages of some antipsychotic drugs, such as clozapine, occupy approximately 36% to 59% of brain dopamine D1 receptors (21), there is no currently known reason to believe that these occupied D1 receptors contribute to the unique properties of clozapine. Third, for the D1 dopamine receptor, the binding constants (that is, the dissociation constants, also referred to as the inhibition constants, or Ki values) of various antipsychotic drugs (22) are very much higher than the concentrations of antipsychotic drugs found in the cerebrospinal fluid or in the plasma water of patients (12, 23, 24). In other words, if the free concentrations of antipsychotic drugs were as high as the values for the binding constants at D1, the drugs would be toxic or lethal to patients.
Of the 3 D2-like receptors, only the D2 receptor itself is blocked by antipsychotic drugs in direct relation to their clinical antipsychotic potencies (8, 9, 25). Although this long-known relation is sometimes criticized as simply a relation between the D2-blocking concentrations and the clinical dosages at which EPS first appear, it is important to note that the concentrations of antipsychotics which block D2 receptors in the brain are precisely identical to the concentrations found in the spinal fluid or plasma water (that is, corrected for drug binding to the plasma proteins) of patients whose psychotic symptoms are successfully controlled by antipsychotics (Figure 1). Because it is known that the clinical efficacy of antipsychotics is associated with a blockade of 60% to 80% of D2 receptors in the brain (26–28), the antipsychotic concentrations shown on the ordinate in Figure 1 are those needed to block 75% of D2 receptors in the presence of a physiological concentration of dopamine (see next section).
Endogenous dopamine raises the antipsychotic concentration needed for D2block
Upon entry into the synaptic space, the antipsychotic drug must compete with endogenous dopamine for the receptor. Thus, the antipsychotic therapeutic concentration needed to block 50% of dopamine receptors in the presence of dopamine will be higher than that needed in the absence of dopamine. This is in accordance with the equation C50% = Ki ′ [1+D/D2high], where D is the dopamine concentration in the synaptic space and where D2high is the dissociation constant of dopamine at the high-affinity state of the dopamine D2 receptor. The level of dopamine in the synaptic space in humans is not known but, in the rat nucleus accumbens, it is between 1 and 4 nM at rest, momentarily rising to 200 nM for the few milliseconds it takes for a nerve impulse to propagate (29). The dopamine D2 receptor can exist in either a high-affinity state or a low-affinity state for dopamine. The high-affinity state, D2high, is the physiologically functional state (30). The dissociation constant of dopamine at D2high is 1.75 nM (see later).
Hence, although the concentration of dopamine, D, in the human synaptic space is not known, it appears that D is of the same order of magnitude as the dopamine Ki for D2high. Hence, with this single assumption that D is equivalent to D2high, the above equation of C50% = Ki ′ [1 + D / D2high] reduces to C50% is equivalent to 2 ′ Ki. The fraction, F, of D2 receptors occupied by an antipsychotic at a concentration C is C / (C + Ki). Using this formula, it can be shown that the concentration of an antipsychotic drug needed to occupy 75% of the D2 receptors is about 3 times higher than that required to occupy 50% of the receptors.
Therefore, using the above equations, the antipsychotic concentrations to occupy 75% of D2 receptors in patients were calculated and found to be virtually identical to the therapeutic concentrations of the antipsychotic drugs in the cerebrospinal fluid or in the plasma water (that is, corrected for drug binding to the plasma proteins) of patients being successfully maintained on these medications. This is illustrated in Figure 1.
“Fast-off” theory of atypical antipsychotic action: atypicals are rapidly released from D2receptors
As noted above, clinically effective dosages of antipsychotic drugs occupy between 60% and 80% of brain dopamine D2 receptors in patients, as measured by positron emission tomography (PET) or single photon emission tomography (SPET) in the human striatum (28, 32–37, 38–53). Clozapine and quetiapine, however, have consistently been apparent exceptions. For example, in patients taking therapeutically effective antipsychotic dosages of clozapine, this drug only occupies between 0% and approximately 50% of brain dopamine D2 receptors, as measured by various radioligands using either PET (26, 28, 31, 34–36, 54–58) or SPET (47–52, 59).
Because the atypical antipsychotics occupy many different types of receptors under therapeutic conditions, any apparent exceptions to the “60% to 80%” rule of D2 occupancy must be taken seriously. For example, the apparently low occupancy of D2 by clozapine might suggest that D2 is not the major antipsychotic target for clozapine (31, 60). This is an important point because, if D2 is not the common target for all antipsychotic drugs, then the explanation for atypicality must be found elsewhere, perhaps in the 5-HT system or in the balance between 5-HT and dopamine.
However, the apparently low occupancy of D2 by clozapine and quetiapine is readily explained by the fact that these 2 antipsychotics rapidly dissociate from the dopamine D2 receptor (27). This also holds for remoxipride and amisulpride, 2 atypical drugs not used clinically in Canada. For example, human-cloned dopamine D2 receptors release [3H]clozapine, [3H]quetiapine, [3H]remoxipride, and [3H]amisulpride at least 100 times faster than they release [3H]haloperidol or [3H]chlorpromazine (27, 61, 62).
Figure 2 shows the rapid release of [3H]clozapine, [3H]quetiapine, [3H]remoxipride, and [3H]amisulpride from human-cloned dopamine D2 receptors; the slow release of [3H]raclopride, [3H]haloperidol, and[3H]chlorpromazine; and the intermediate release rates from these receptors for [3H]olanzapine and [3H]sertindole.
These in vitro data match those found clinically for clozapine, quetiapine, and haloperidol in schizophrenia patients and healthy volunteers. This is shown in Figure 3, where it has been found by PET (using [11C]raclopride) that the human brain (striatum) occupancy of D2 by quetiapine and clozapine rapidly falls off within 24 hours, in contrast to that for haloperidol, which maintains its D2 occupancy constant over 24 hours (38, 53, 63).
Thus, the rapid release of clozapine and quetiapine from dopamine D2 receptors and their replacement by endogenous dopamine would readily account for the low D2 receptor occupancy shown by these atypical antipsychotics.
It is important to emphasize that the rapid release of clozapine and quetiapine is a molecular event which occurs quickly, regardless of the clinical dosage used. In other words, even though high dosages of clozapine and quetiapine may be used, these drugs continue to go on and off the D2 receptor rapidly, allowing extensive and frequent access of endogenous dopamine to the receptor.
Hence, it appears that some antipsychotics, such as clozapine and quetiapine, occupy D2 receptors only transiently throughout the day. As just mentioned, PET imaging of patients with schizophrenia reveals that the D2 receptor occupancies by clozapine and quetiapine wear off quickly after an oral dosage, and patients may show no occupancy whatsoever within 48 hours of the last dose, in contrast to typical antipsychotics, which may continue to occupy D2 receptors for days. This may explain why psychotic relapses of patients on clozapine and quetiapine occur soon after withdrawal of the antipsychotic (64, 65, reviewed in 27)—much earlier than after withdrawal of conventional antipsychotic drugs such as haloperidol or chlorpromazine.
Clinical and basic implications of the “fast-off” theory of atypical antipsychotic action
As outlined above, the “fast-off” theory of atypical antipsychotic action is that the atypicals have low affinities for the dopamine D2 receptor, and are loosely bound to, and rapidly released from, these receptors. A critical aspect of the theory is that the atypical antipsychotics bind more loosely to D2 than does dopamine it self, while the traditional, typical antipsychotics bind more tightly than dopamine. These data are summarized in Table 1 and Figure 4A.
Figure 4A illustrates a general demarcation between typicals and atypicals. That is, the typical antipsychotics have K values lower than that for dopamine (at the high-affinity state of the D2 receptor), while the atypicals have K values higher than that for dopamine. Although risperidone appears to be an exception to this generalization, risperidone is the weakest “atypical” antipsychotic, eliciting dosage-dependent EPS in 60% to 70% of patients taking 6 mg or more daily, a dosage that may be insufficient for clinical efficacy (66).
Clearly, the separation between typicals and atypicals in Figure 4A is not sharp and precise, because antipsychotic drugs with K values between 2 nM and 10 nM (including molindone and loxapine) often reveal dose-dependent EPS. Thus, the demarcation between typical and atypical antipsychotics is not a sharp divide but rather a continuous one. Antipsychotics become increasingly more atypical as their binding to the D2 receptor becomes more loose and they are released more quickly. Although all atypical antipsychotics have loose binding, with dissociation constants looser than 1.8 nM/L, they can still elicit dosage-dependent parkinsonism. For example, olanzapine, with a dissociation constant of 5.1 nM, is known to be associated with a dose-dependent incidence of EPS in some patients and especially at higher dosages. If the binding is extremely loose, as with clozapine, remoxipride, quetiapine, and melperone, essentially no EPS occurs (although exquisitely sensitive patients do exist who will exhibit EPS even with these drugs). Drugs that are too loose or have far too low an affinity for D2 receptors cease to exhibit any antipsychotic activity at all. Moreover, although the degree of occupancy of atypicals at D2 receptors has a direct influence on EPS, the potent anticholinergic action of olanzapine and clozapine provides an additional anti-EPS mechanism. It is because of its anticholinergic properties, for instance, that thioridazine use is relatively free of EPS.
Table 2 summarizes a few clinical distinctions between the typical antipsychotics, which are tightly bound to D2, and the atypical antipsychotics, which are loosely bound to D2. The required antipsychotic dosage (in mg) will be low for tightly bound drugs but high for loosely bound drugs. The typicals, being tightly bound to D2, will elicit EPS and elevated prolactin, while the atypicals, being loosely bound and rapidly released from D2, will not elicit these side effects, or will at least elicit them to a markedly lesser extent. Finally, because the typicals remain attached to D2 and readily accumulate in brain tissue, they will eventually lead to TD (67). The atypicals, however, are much less fat-soluble, and because they are readily released from D2 and from the brain tissue, the risk of causing TD is much reduced or perhaps absent.
l-Dopa psychosis: “fast-off-D2” theory predicts low dosage of atypical antipsychotics
The treatment of patients with psychosis in Parkinson’s disease (as a consequence of l-dopa treatment) is best done with a very loose binding antipsychotic, such as clozapine or quetiapine, to allow for the low level of dopamine neurotransmission that is required for normal motor functioning to continue. Parkinson patients are dopamine-depleted, so it is very important not to block the little dopamine function that remains. The hypothesis is that atypical antipsychotic action (that is, low EPS and normal prolactin) occurs when endogenous dopamine is able to displace a loosely bound antipsychotic. This accords with the observation that low dosages of atypical antipsychotics are useful for Parkinson patients’.
It is well known in neurology that l-dopa psychosis in a patient with Parkinson’s disease is best treated with a dosage of clozapine that is about 10% the dosage normally used for psychosis in schizophrenia. The “fast-off-D2” hypothesis readily and quantitatively predicts this. As presented above, the antipsychotic dosage needed to occupy D2 receptors is proportional to K ′ [1 + D / Dhigh], where K is the dissociation constant of the antipsychotic, D is the concentration of dopamine in the synaptic space during the momentary nerve impulse (equivalent to 200 nM, ref. 29), and where Dhigh is the dissociation constant of dopamine at the high-affinity state of D2 (equivalent to 1.75 nM; Table 1). In Parkinson’s disease, where 90% to 95% of the dopamine content is absent, the value for D would be equivalent to 20 nM. Accordingly, the antipsychotic dosage for l-dopa psychosis will be lower than that for schizophrenia psychosis by a factor of (1 + D / Dhigh) normal / (1 + D / Dhigh) Parkinson, or (1 + 200 / 1.75) / (1 + 20 / 1.75), or tenfold. Thus, while a daily dosage of 500 mg clozapine might be suitable for treating schizophrenia psychosis, a dosage of 50 mg (or less) would be more than adequate to treat l-dopa psychosis. It is important to note that this calculation best holds for competition between endogenous dopamine and a loosely bound antipsychotic. A tightly bound antipsychotic such as haloperidol would not readily permit endogenous dopamine to replace it competitively.
Test of the “fast-off-D2” hypothesis using clozapine and isoclozapine
As reported by Kapur and others (68), the single most powerful predictor of atypicality is the low affinity to, and fast dissociation from, the D2 receptor—not high affinity to any other receptor. This hypothesis is supported by their findings that clozapine and isoclozapine have identical potencies on many cloned receptors (including muscarinic M1, dopamine D1, dopamine D4, 5-HT1A, and 5-HT2A receptors) but differ fivefold in their potency only on D2 receptors. Thus, in several tests of atypicality (for example, early activation of certain genes, catalepsy in animals, and prolactin elevation), clozapine behaves like an atypical antipsychotic. Isoclozapine, however, behaves like a conventional antipsychotic.
Do antipsychotics elicit atypical action by blocking 5-HT receptors?
In addition to blocking dopamine receptors, the new atypical antipsychotic drugs also block 5-HT receptors. Although it has of ten been suggested that the blockade of 5-HT2A receptors may alleviate the parkinsonism caused by D2 blockade (69, 70), most data do not support this principle.
Remoxipride is an important exception
Remoxipride is a highly effective atypical antipsychotic drug (not used in Canada) with no EPS and no hyperprolactinemia, yet it does not block 5-HT receptors.
5-HT blockade enhances catalepsy
Selective 5-HT2A receptor blockade with the drug M100,907 markedly enhances, instead of reducing, the catalepsy (catalepsy in animals = EPS in humans) observed with submaximal dosages of the D2 block by raclopride (71).
No dopamine–5-HT correlation to cataleptic dosages
There is no correlation between the cataleptic dosages of neuroleptics and the ratio of the antipsychotic dissociation constants at D2 and at 5-HT2A receptors (72, 73).
No sharp separation of typicals and atypicals
Using the ratio of antipsychotic dissociation constants obtained in our laboratory on human-cloned D2 and 5-HT2A receptors (Table 1), the demarcation between typical and atypical antipsychotics shown in Figure 4B is not sharp and is less clear than that found in Figure 4A. For example, of the 20 antipsychotics in Figure 4B, there are 3 to 4 apparent exceptions to the separation of typicals and atypicals. This compares to 2 or 3 apparent exceptions out of the 31 antipsychotics shown in Figure 4A. (Exceptions do not necessarily kill a theory, but explanations for the exceptions have to be found.)
No alleviation of extrapyramidal signs
A high degree of 5-HT2A receptor occupancy (95%) by risperidone (6 mg daily) does not prevent EPS in 6 out of 7 patients (74, 75).
5-HT block does not change D2occupancies required
Using [11C]raclopride for imaging brain D2 receptors and [11C]setoperone for imaging brain 5-HT2A receptors, Kapur and others found that the high occupancy of 5-HT2A receptors by olanzapine or by risperidone did not alter either the D2 occupancy required for the antipsychotic effect or the D2 occupancy at which EPS occur (28). The threshold dosages for antipsychotic action consistently occupy 65% of brain D2 receptors in patients, and the threshold dosages for EPS consistently occupy 80% of brain D2 receptors in patients, whether or not the 5-HT2A receptors are occupied. As illustrated in Figure 5, the results showed that the occupancy of D2 receptors in first-episode schizophrenia patients was 65% for antipsychotic threshold dosages of haloperidol (1.5 to 2.1 mg daily) and olanzapine (7.5 to 10 mg daily), despite the negligible occupancy of 5-HT2A receptors by haloperidol or the very high occupancy, exceeding 95%, by olanzapine. It is important to note that while first-episode patients may not be typical of patients with chronic schizophrenia, studies with the first-episode patients are exceedingly important in working out mechanisms of antipsychotic action, because they have had not previous exposure to drugs. It is not clear what clinical benefit, if any, is provided by the blockade of 5-HT receptors. Although low dosages of cyproheptadine have been used (64) to block 5-HT2A receptors and supplement antipsychotic administration, it should be noted that cyproheptadine has a D2 blocking action. It has a K of 24 nM at D2 receptors, compared with 63 nM for clozapine and 21 nM for amoxapine (Table 1). Amoxapine, though marketed as an antidepressant, has antipsychotic properties.
Amisulpride is an important exception
Amisulpride (used in Europe) is a highly effective antipsychotic that is atypical and does not occupy any 5-HT2A receptors in humans at dosages up to 1200 mg daily (76).
Chlorpromazine blocks 5-HT but elicits EPS
Chlorpromazine, the first typical antipsychotic, blocks 65% of 5-HT2A receptors at 500 mg daily. This “high level of 5-HT2A block . . . suggests that the distinct clinical profiles of chlorpromazine and clozapine are unrelated to 5-HT2A receptor blockade” (76).
5-HT block not needed for antipsychotic action
It has also been stated that the block of 5-HT2A receptors “is not a prerequisite for the antipsychotic effect” (74, 75). In fact, full block of 5-HT2A receptors occurs at subtherapeutic dosages of risperidone, olanzapine, and clozapine, indicating that 5-HT2A block has little or no antipsychotic action.
Do antipsychotics elicit atypical action by stimulating 5-HT receptors?
Although it has long been known that the stimulation of 5-HT1A receptors in animals can alleviate catalepsy caused by D2 blockade (77, 78), there do not appear to be any antipsychotics that have this 5-HT1A-stimulating action combined with D2-blocking action.
It has recently been proposed that the stimulation of 5-HT2A receptors by an inverse action is an important contribution to atypical antipsychotic action (79). This is illustrated in Figure 4C, where the inverse stimulating potencies of antipsychotics on 5-HT2A receptors are shown (from 79). However, because a few important atypical antipsychotics (including remoxipride and sulpiride) have no such stimulating action, it is unlikely that this feature contributes to atypical antipsychotic action. The data in Figure 4C do not reveal any clear demarcation between typicals and atypicals. Finally, Although the authors (79) propose that M100,907 has the desired stimulating action, this compound has shown no antipsychotic activity in humans.
The future
Because brain imaging indicates that the traditional antipsychotics remain attached to dopamine D2 receptors for at least 1 or 2 days, there is no rational need to medicate schizophrenia patients daily with typical antipsychotics. This reasoning has led to a new regimen of administering antipsychotics by “extended dosing,” wherein the patient receives a typical antipsychotic once every 3rd or 4th day (80). Such a procedure, of course, could not be used with atypical antipsychotics because of their loose binding to D2 and subsequent risk of relapse.
Clinicians can now apply this knowledge to the treatment of individual patients. Atypical agents, being newer and still protected by patent, are much more expensive than the older drugs. The fact that they do not elicit EPS and do not elevate prolactin levels does not mean that they are free of serious side effects. One could argue that the side effects associated with some of the atypical drugs (for example, agranulocytosis, obesity, diabetes, ophthalmological problems, cardiovascular problems, sexual problems, obsessive-compulsive symptoms, convulsions, and insomnia) are more serious than EPS, high prolactin, and even TD. Low-dose, extended-dosing regimens of typical drugs may be best suited for specific patients. Patients known to be nonadherent to regular medication may do better on those drugs that are more tightly bound to the D2 receptor, where risk of relapse through a short period of noncompliance is reduced. Conversely, patients with a history of neuroleptic malignant syndrome are best treated with drugs that are readily displaced, so that, should the syndrome return, the drug is quickly out of their brain. In patients with psychosis, high stress levels, accompanied by high endogenous dopamine release, will necessitate higher dosages of the antipsychotic drug. Periods of low stress will require lower dosages. Patients with psychosis who may temporarily benefit from high prolactin levels (for example, those who do not want to conceive or, conversely, postpartum women whose milk is in sufficient for breastfeeding) may preferentially be prescribed typical antipsychotics. On the other hand, typical antipsychotics should be discontinued in those with beginning signs of TD and the newer drugs prescribed instead. Knowing how drugs work greatly expands the clinician’s repertoire of strategies, allowing optimization of drug regimens for individualized treatment.
K Value, nM Dopamine D2 Receptora | K Galue, nM 5-HT2a Receptorb | Ratio: ![]() | K Value, nM 5-HT2A Receptorc | |
---|---|---|---|---|
M100,907 (not antipsychotic) | 9000 | 0.2 | 45,000 | 0.3 |
Melperone (atypical) | 152 | 180 | 0.84 | 110 |
Perlapine (atypical) | 138 | 22 | 6.3 | 30 |
Quetiapine (atypical) | 122d | 135 | 0.9 | 270 |
Remoxipride (atypical) | 67 | 6600 | 0.01 | no effect |
Clozapine (atypical) | 63e | 3.7m | 17 | 6.4 |
Amoxapine (atypical) | 21 | 0.6 | 35 | 3.6 |
Sulpiride-S (atypical) | 9.9 | no effect | ||
Loxapine (typical) | 9.6 | 2 | 4.8 | 4.2 |
Iloperidone (atypical) | 5.4 | 0.2 | 27 | |
Olanzapine (atypical) | 5.1f | 2.5m | 2 | 6.8 |
Molindone (typical) | 4.9 | 5200 | 0.001 | no effect |
Ziprasidone (atypical) | 2.7 | 3 | 0.9 | |
Sertindole (atypical) | 2.3g | 0.28m | 8.2 | 0.1 |
Amisulpride-S (atypical) | 1.8h | |||
Dopamine at D2high | 1.75n | |||
Raclopride (typical) | 1.7i | 4400 | 4E-04 | |
Prochlorperazine (typical) | 1.7 | |||
Moperone (typical) | 1.6 | 87 | ||
Pimozide (typical) | 1.4 | 2.2 | ||
Trifluperazine (typical) | 1.4 | 8.8 | 0.16 | 130 |
Risperidone (atypical?) | 1.1 | 0.2 | 5.5 | 1.8 |
Thioridazine (typical) | 1.1 | 1.3 | 0.85 | 100 |
Chlorpromazine (typical) | 0.99j | 2 | 0.5 | 16 |
Chlorprothixene (typical) | 0.7 | |||
Haloperidol (typical) | 0.55k | 60 | 0.009 | 210 |
Fluphenazine (typical) | 0.55 | 3.8 | 0.15 | 17 |
Droperidol (typical) | 0.54 | |||
Flupentixol-cis (typical) | 0.38 | 7 | ||
Perphenazine (typical) | 0.27 | |||
Thiothixene-cis (typical) | 0.15 | 320 | ||
Butaclamol-(+) (typical) | 0.14 | 14 | ||
Spiperone (typical) | 0.04l | 0.57 | 0.07 | 1.6 |
Epidepride (typical) | 0.036 | |||
Nemonapride (typical) | 0.014 | 7 |
Tight | Loose | |
---|---|---|
Dosage | Low | High |
Extrapyramidal symptoms (EPS) | Yes | No |
Prolactin | High | Normal |
Tardive Dyskinesia | High risk | Low risk |
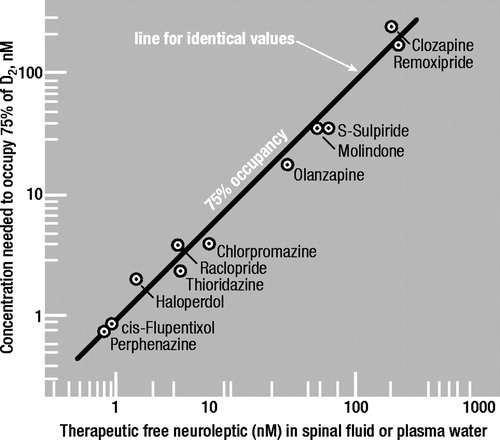
Figure 1. The concentrations of antipsychotic drugs that block dopamine D2 receptors in vitro (using [3H]raclopride) are identical to the concentrations of antipsychotic drugs that are found in the spinal fluid or in the plasma water (that is, corrected for drug binding to the plasma proteins) of patients being successfully maintained on these drugs. The antipsychotic concentrations needed to block 75% of D2 receptors in vitro is shown (ordinate) because it is known that the clinical action of antipsychotics is associated with a block of 60% to 80% of D2 receptors. (See text for additional details.)
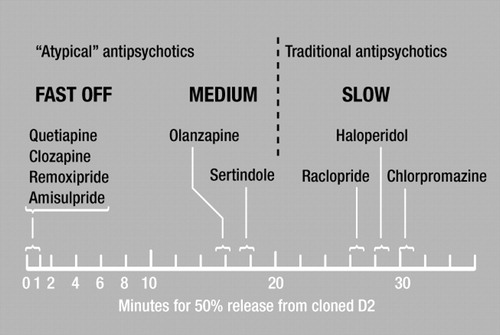
Figure 2. Human cloned D2 receptors were equilibrated with the tritium-labelled antipsychotic drug, after which a high concentration of raclopride or dopamine was used to displace the antipsychotic drug. The typical antipsychotic drugs chlorpromazine, haloperidol, and raclopride dissociated slowly over 30 minutes, while the atypical antipsychotics dissociated rapidly in under 60 seconds. Olanzapine and sertindole had intermediate rates of dissociation. (See refs. 61, 62 for details.) The final concentration of a clopride was 100 micromolar, in contrast to that of 10 micromolar used earlier (ref. 61), explaining why the 50% off set times for radioactive haloperidol, or radioactive sertindole, were lower than the previously published data (ref. 61).
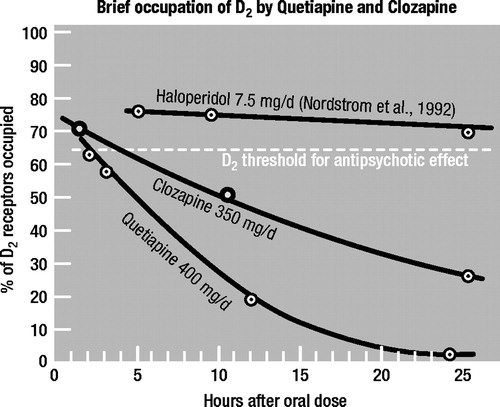
Figure 3. Positron emission tomography imaging (using [11C]raclopride) reveals that the human brain (striatum) occupancy of D2 by quetiapine and clozapine rapidly falls off within 24 hours, in contrast to that for haloperidol, which maintains its D2 occupancy constant over 24 hours (adapted from refs. 38, 53, 63).
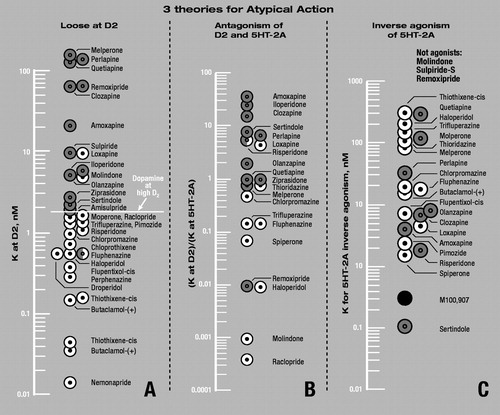
Figure 4A, 4B, 4C. Comparing 3 theories for atypical antipsychotic action. Typical antipsychotics are unshaded, atypicals are shaded. Risperidone is a weak atypical, and is half shaded, half unshaded. A: The “fast-off-D2” theory proposes that typical antipsychotics bind more tightly than dopamine to the dopamine D2 receptor (in its functional high-affinity state), with dissociation constants lower than that for dopamine, while the atypicals bind more loosely than dopamine to the dopamine D2 receptor, with dissociation constants higher than that for dopamine. Out of 31 antipsychotics, there are 2 or 3 apparent exceptions to this rule. Drugs with K values between 2 and 10 nM cause dose-dependent extrapyramidal signs. B: The dopamine-serotonin antagonism theory generally predicts a separation between typicals and atypicals, except that out of 20 antipsychotics there are 3 or 4 apparent exceptions to this theory. Remoxipride is an important exception. C: The theory which predicts that antipsychotics stimulate 5-HT2A receptors by inverse agonism has many exceptions, including M100,907, which has no antipsychotic action.
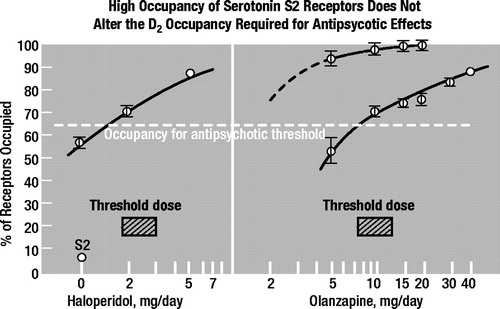
Figure 5. The threshold doses for antipsychotic action consistently occupy 65% of brain D2 receptors in patients, and the threshold doses for extrapyramidal signs consistently occupy 80% of brain D2 receptors in patients, whether or not the 5-HT2A receptors are occupied. As shown here, the occupancy of D2 receptors in first-episode schizophrenia patients was 65% for antipsychotic threshold doses of haloperidol (1.5 to 2.1 mg daily) and olanzapine (7.5 to 10 mg daily), despite the negligible occupancy of 5-HT2A receptors by haloperidol or the very high occupancy, exceeding 95%, by olanzapine. (Adapted from ref. 28).
1 Delay J, Deniker P, Harl J-M. Traitement des états d’excitation et d’agitation par une méthode médicamenteuse dérivée de l’hibernothérapie. Ann Méd Psychologie 1952; 110 (Part 2):267–73 Google Scholar
2 Haase HJ, Janssen PAJ. The action of neuroleptic drugs: a psychiatric, neurologic and pharmacological investigation. Chicago: Year Book Medical Publ; 1965 Google Scholar
3 Van Rossum J. The significance of dopamine-receptor blockade for the action of neuroleptic drugs. In: Brill H, Cole J, Deniker P, Hippius H, Bradley PB, editors. Neuropsychopharmacology, Proceedings 5th Collegium Internationale Neuropsychopharmacologicum. Amsterdam: Excerpta Medica; 1967. p 321–9 Google Scholar
4 Carlsson A, Lindqvist M. Effect of chlorpromazine or haloperidol on formation of 3-methoxytyramine and normetanephrine in mouse brain. Acta Pharmacol Toxicol 1963; 20:140–4 Crossref, Google Scholar
5 Andén N-E, Roos B-E, Werdinius B. Effects of chlorpromazine, haloperidol and reserpine on the levels of phenolic acids in rabbit corpus striatum. Life Sci 1964; 3:149–58 Crossref, Google Scholar
6 Andén N-E, Butcher SG, Corrodi H, Fuxe K, Ungerstedt U. Receptor activity and turnover of dopamine and noradrenaline after neuroleptics. Eur J Pharmacol 1970; 11:303–14 Crossref, Google Scholar
7 Seeman P, Wong M, Lee T. Dopamine receptor-block and nigral fiber-impulse blockade by major tranquilizers. Fed Proc 1974; 33:246 Google Scholar
8 Seeman P, Chau-Wong M, Tedesco J, Wong K. Brain receptors for antipsychotic drugs and dopamine: direct binding assays. Proc Natl Acad Sci USA 1975; 72:4376–80 Crossref, Google Scholar
9 Seeman P, Lee T, Chau-Wong M, Wong K. Antipsychotic drug doses and neuroleptic/dopamine receptors. Nature 1976; 261:717–9 Crossref, Google Scholar
10 Pickar D. Prospects for the pharmacotherapy of schizophrenia. Lancet 1995; 345:557–62 Crossref, Google Scholar
11 Su T-P, Malhotra AK, Hadd K, Breier A, Pickar D. D2 dopamine receptor occupancy: a crossover comparison of risperidone with clozapine therapy in schizophrenic patients. Arch Gen Psychiatry 1997; 54:972–3 Crossref, Google Scholar
12 Seeman P. Dopamine receptor sequences. Therapeutic levels of neuroleptics occupy D2, clozapine occupies D4. Neuropsychopharmacology 1992; 7:261–84 Google Scholar
13 Seeman P, Corbett R, Nam D, Van Tol HHM. Dopamine and serotonin receptors: amino acid sequences, and clinical role in neuroleptic parkinsonism. Jpn J Pharmacol 1996; 71:187–204 Crossref, Google Scholar
14 Sunahara RK, Niznik HB, Weiner DM, Stormann TM, Brann MR, Kennedy JL, and others. Human dopamine D1 receptor encoded by an intronless gene on chromosome 5. Nature 1990; 347:80–3 Crossref, Google Scholar
15 Sunahara RK, Guan H-C, O’Dowd BF, Seeman P, Laurier LG, Ng GYK, and others. Cloning of the gene for a human dopamine D5 receptor with higher affinity for dopamine than D1. Nature 1991; 350:614–9 Crossref, Google Scholar
16 Lidow MS, Williams GV, Goldman-Rakic PS. The cerebral cortex: a case for a common site of action of antipsychotic drugs. Trends Pharmacol Sci 1998; 19:136–40 Crossref, Google Scholar
17 Karlson P, Smith L, Farde L, Härnryd C, Sedvall G, Wiesel F-A. Lack of apparent antipsychotic effect of the D1-dopamine receptor antagonist SCH39166 in acutely ill schizophrenic patients. Psychopharmacology 1995; 121:309–16 Crossref, Google Scholar
18 Den Boer JA, van Megen HJGM, Fleischacker WW, Louwerens JW, Slaap BR, Westenberg HGM, and others. Differential effects of the D1-DA receptor antagonist SCH39166 on positive and negative symptoms of schizophrenia. Psychopharmacology 1995; 121:317–22 Crossref, Google Scholar
19 De Beaurepaire R, Labelle A, Naber D, Jones BD, Barnes TRE. An open trial of the D1 antagonist SCH39166 in six cases of acute psychotic states. Psychopharmacology 1995; 121:323–7 Crossref, Google Scholar
20 Farde L, Nordström A-L. PET analysis indicates atypical central dopamine receptor occupancy in clozapine-treated patients. Br J Psychiatry 1992; 160 (Suppl 17):30–3 Crossref, Google Scholar
21 Nordström A-L, Farde L, Nyberg S, Karlsson P, Halldin C, Sedvall G. D1, D2, and 5-HT2 receptor occupancy in relation to clozapine serum concentration: A PET study of schizophrenic patients. Am J Psychiatry 1995; 152:1444–9 Crossref, Google Scholar
22 Seeman P, Niznik HB. Dopamine D1 receptor pharmacology. ISI Atlas of Sci Pharmacology 1988; 2:161–70 Google Scholar
23 Seeman P. Dopamine receptors and the dopamine hypothesis of schizophrenia. Synapse 1987; 1:133–52 Crossref, Google Scholar
24 Seeman P, Tallerico T. Antipsychotic drugs which elicit little or no Parkinsonism bind more loosely than dopamine to brain D2 receptors, yet occupy high levels of these receptors. Mol Psychiatry 1998; 3:123–34 Crossref, Google Scholar
25 Creese I, Burt DR, Snyder SH. Dopamine receptor binding predicts clinical and pharmacological potencies of antischizophrenic drugs. Science 1976; 192:481–3 Crossref, Google Scholar
26 Farde L, Nordström A-L, Wiesel F-A, Pauli S, Halldin C, Sedvall G. Positron emission tomographic analysis of central D1 and D2 dopamine receptor occupancy in patients treated with classical neuroleptics and clozapine. Relation to extrapyramidal side effects. Arch Gen Psychiatry 1992; 49:538–44 Crossref, Google Scholar
27 Seeman P, Tallerico T. Rapid release of antipsychotic drugs from dopamine D2 receptors: an explanation for low receptor occupancy and early clinical relapse upon drug withdrawal of clozapine or quetiapine. Am J Psychiatry 1999; 156:876–84 Crossref, Google Scholar
28 Kapur S, Zipursky RB, Remington G. Comparison of the 5-HT2 and D2 receptor occupancy of clozapine, risperidone, and olanzapine in schizophrenia: clinical and theoretical implications. Am J Psychiatry 1999; 156:286–93 Google Scholar
29 Kawagoe KT, Garris PA, Wiedemann DJ, Wightman RM. Regulation of transient dopamine concentration gradients in the microenvironment surrounding nerve terminals in the rat striatum. Neurosci ence 1992; 51:55–64 Crossref, Google Scholar
30 George SR, Watanabe M, Di Paolo T, Falardeau P, Labrie F, Seeman P. The functional state of the dopamine receptor in the anterior pituitary is in the high-affinity form. Endocrinology 1985; 117:690–7 Crossref, Google Scholar
31 Farde L, Hall H. Positron emission tomography—examination of chemical transmission in the living human brain. Arzneim-Forsch 1992; 42:260–4 Google Scholar
32 Nyberg S, Nordström A-L, Halldin C, Farde L. Positron emission tomography studies on D2 dopamine receptor occupancy and plasma antipsychotic drug levels in man. Int Clin Psychopharmacol 1995; 10 (Suppl 3):81–5 Google Scholar
33 Kapur S, Remington G, Zipursky RB, Wilson AA, Houle S. The D2 dopamine receptor occupancy of risperidone and its relationship to extrapyramidal symptoms: a PET study. Life Sci 1995; 57:PL103–PL107 Crossref, Google Scholar
34 Kapur S, Remington G, Jones C, Wilson A, DaSilva J, Houle S, Zipursky RB. High levels of dopamine D2 receptor occupancy with low-dose haloperidol treatment: a PET study. Am J Psychiatry 1996; 153:948–50 Crossref, Google Scholar
35 Kapur S, Zipursky RB, Jones C, Remington GJ, Wilson AA, DaSilva J, and others. The D2 receptor occupancy profile of loxapine determined using PET. Neuropsychopharmacology 1996; 15:562–6 Crossref, Google Scholar
36 Kapur S, Zipursky RB, Remington G, Jones C, DaSilva J, Wilson AA, and others. 5-HT2 and D2 receptor occupancy of olanzapine in schizophrenia: a PET investigation. Am J Psychiatry 1998; 155:921–8 Crossref, Google Scholar
37 Knable MB, Heinz A, Coppola R, Gorey J, Weinberger DR. IBZM SPECT measurement of D2 receptor occupancy by haloperidol and risperidone. Biol Psychiatry 1996; 39:515 Crossref, Google Scholar
38 Farde L, Wiesel FA, Nordström A-L, Sedvall G. D1 and D2-dopamine receptor occupancy during treatment with conventional and atypical neuroleptics. Psychopharmacology 1989; 99:S28–S31 Crossref, Google Scholar
39 Tauscher J, Küfferle B, Barnas C, Asenbaum S, Brücke T, Kasper S. Dopamine-2 receptor imaging in psychotic patients treated with quetiapine, clozapine, risperidone and haloperidol. Biol Psychiatry 1997; 42:15S Crossref, Google Scholar
40 Küfferle B, Tauscher J, Asenbaum S, Vesely C, Podreka I, BrückeT, and others. IBZM SPECT imaging of striatal dopamine-2 receptors in psychotic patients treated with the novel antipsychotic substance quetiapine in comparison to clozapine and haloperidol. Psychopharmacology 1997; 133:323–8 Crossref, Google Scholar
41 Nordström A-L, Farde L, Wiesel F-A, Forslund K, Pauli S, Halldin C, and others. Central D2-dopamine receptor occupancy in relation to antipsychotic drug effects: a double-blind PET study of schizophrenic patients. Biol Psychiatry 1993; 33:227–35 Crossref, Google Scholar
42 Miceli JJ, Gunn KP, Ru bin RH, Frackowiak RSJ, Wil liams SA, Fischman A. 5HT2 and D2 receptor occupancy of ziprasidone in healthy volunteers. Am Coll Neuropsychopharmacol 1996; 35:269 Google Scholar
43 Bench CJ, Lammertsma AA, Grasby PM, Dolan RJ, Warrington SJ, Boyce M, and others. The time course of binding to striatal dopamine D2 receptors by the neuroleptic ziprasidone (CP-88,059-01) determined by positron emission tomography. Psychopharmacology 1996; 124:141–7 Crossref, Google Scholar
44 Kasper S, Tauscher J, Küfferle B, Asenbaum S, Barnas C, Hesselmann B, and others. Sertindole and dopamine D2 receptor occupancy. Biol Psychiatry 1997; 42:161S Crossref, Google Scholar
45 Kasper S, Tauscher J, Küfferle B, Barnas C, Hesselmann B, Asenbaum S, and others. Sertindole and dopamine D2 receptor occupancy in comparison to risperidone, clozapine and haloperidol. Psychopharmacology 1998; 136:367–73 Crossref, Google Scholar
46 Kapur S, Zipursky R, Remington G, Jones C, McKay G, Houle S. PET evidence that loxapine is an equipotent blocker of 5-HT2 and D2 receptors: Implications for the therapeutics of schizophrenia. Am J Psychiatry 1997; 154:1525–9 Crossref, Google Scholar
47 Busatto GF, Pilowsky LS, Costa DC, Ell PJ, Verhoeff NPLG, Kerwin RW. Dopamine D2 receptor blockade in vivo with the novel antipsychotics risperidone and remoxipride—an 123I-IBZM single photon emission tomography (SPET) study. Psychopharmacology 1995; 117:55–61 Crossref, Google Scholar
48 Klemm E, Grünwald F, Kasper S, Menzel C, Broich K, Danos P, and others. [123I]IBZM SPECT for imaging of striatal D2 dopamine receptors in 56 schizophrenic patients taking various neuroleptics. Am J Psychiatry 1996; 153:183–90 Crossref, Google Scholar
49 Scherer J, Tatsch K, Schwarz J, Oertel WH, Konjarczyk M, Albus M. D2-dopamine receptor occupancy differs between patients with and without extrapyramidal side effects. Acta Psychiatr Scand 1994; 90:266–8 Crossref, Google Scholar
50 Pilowsky LS, Busatto GF, Taylor M, Costa DC, Sharma T, Sigmundsson T, and others. Dopamine D2 receptor occupancy in vivo by the novel atypical antipsychotic olanzapine—a 123I IBZM single photon emission tomography (SPET) study. Psychopharmacology 1996; 124:148–53 Crossref, Google Scholar
51 Su T-P, Breier A, Coppola R, Hadd K, Elman I, Adler C, and others. D2 receptor occupancy in risperidone and clozapine-treated schizophrenics. Biol Psychiatry 1996; 39:512–3 Crossref, Google Scholar
52 Su T-P, Breier A, Coppola R, Hadd K, Elman I, Alder C, and others. D2 receptor occupancy during risperidone and clozapine treatment in chronic schizophrenia: relationship to blood level, efficacy and EPS. Soc Neurosci Abstr 1996; 22:265 Google Scholar
53 Gefvert O, Lundberg T, Wieselgren I-M, Hagström P, Bergström M, Långström B, and others. D2 and 5-HT2A receptor binding of different doses of quetiapine in schizophrenics: a PET study. Am Coll Neuropsychopharmacol 1997; 36:168 Google Scholar
54 Karbe H, Wienhard K, Hamacher K, Huber M, Herholz K, Coenen HH, and others. Positron emission tomography with (18F) methylspiperone demonstrates D2 dopamine receptor binding differences of clozapine and haloperidol. J Neural Transm 1991; 86:163–73 Crossref, Google Scholar
55 Louwerens JW, Buddingh JA, Zijlstra S, Pruim J, Korf J, Paans AMJ, and others. Dopamine (D2)-receptor occupancy in clozapine-treated patients as measured by positron emission tomography using 18FESP. In: Brunello N, Mendlewicz J, Racagni G, editors. New generation of antipsychotic drugs: novel mechanisms of action. Volume 4. Basel: Karger; 1993. p 130–5 Google Scholar
56 Louwerens JW, Slooff CJ, Korf J, Coppens HJ, Paans AMJ. Dopamine2- and serotonin2-receptor-antagonism by antipsychotics in man. Schizophr Res 1996; 18:141 Crossref, Google Scholar
57 Conley R, Medoff D, Wong D, Tamminga C. 11C NMSP receptor occupancy by clozapine and haloperidol in schizophrenic subjects. Schizophr Res 1995; 15:80 Crossref, Google Scholar
58 Conley R, Zhao M, Wong D, Tamminga C. 11C NMSP receptor occupancy by clozapine and haloperidol in schizophrenic subjects. Biol Psychiatry 1996; 39:513 Crossref, Google Scholar
59 Pickar D, Su T-P, Weinberger DR, Coppola R, Malhotra AK, Knable MB, and others. Individual variation in D2 dopamine receptor occupancy in clozapine-treated patients. Am J Psychiatry 1996; 153:1571–8 Crossref, Google Scholar
60 Brunello N, Masotto C, Steardo L, Markstein R, Racagni G. New insights into the biology of schizophrenia through the mechanism of action of clozapine. Neuropsychopharmacology 1995; 13:177–213 Crossref, Google Scholar
61 Kapur S, Seeman P. Antipsychotic agents differ in how fast they come off the dopamine D2 receptors. Implications for atypical antipsychotic action. J Psychiatry Neurosci 2000; 25:161–6 Google Scholar
62 Kapur S, Seeman P. Does fast dissociation from the dopamine D2 receptor explain the action of atypical antipsychotics? A new hypothesis. Am J Psychiatry 2001; 158:360–9 Crossref, Google Scholar
63 Kapur S, Zipursky R, Jones C, Shammi CS, Remington G, Seeman P. A positron emission tomography study of quetiapine in schizophrenia—a preliminary finding of an antipsychotic effect with only transiently high dopamine D2 receptor occupancy. Arch Gen Psychiatry 2000; 57:553–9 Crossref, Google Scholar
64 Meltzer HY, Lee MA, Ranjan R, Ma son EA, Cola PA. Relapse following clozapine withdrawal: Effect of neuroleptic drugs and cyproheptadine. Psychopharmacology (Berl) 1996; 124:176–87 Crossref, Google Scholar
65 Thyrum PT, Yeh C, Potkin SG, and others. Safety and tolerability of switching from conventional antipsychotic therapy to “Seroquel” (quetiapine fumarate) followed by abrupt withdrawal from “Seroquel.” Am College Neuropsychopharmacol 1997; 36:293 Google Scholar
66 Chouinard G, Kopala L, Labelle A, Beauclair L, Johnson SV, Singh KI. Phase-IV multicentre clinical study of risperidone in the treatment of outpatients with schizophrenia. The RIS-CAN-3 study group. Can J Psychiatry 1998; 43:1018–25 Crossref, Google Scholar
67 Seeman P. Tardive dyskinesia, dopamine receptors, and neuroleptic damage to cell membranes. J Clin Psychopharmacol 1988; 8:3S–9S Crossref, Google Scholar
68 Kapur S, Seeman P, Zipursky R, Remington GJ. Fast dissociation from the dopamine D2 receptor (not high affinity at multiple receptors) is the key to “atypical” antipsychotics. Schizophr Res 2001; 49 (Suppl 1,2):92 Google Scholar
69 Meltzer HY, Matsubara S, Lee J-C. Classification of typical and atypical antipsychotic drugs on the basis of dopamine D-1, D-2 and serotonin2 pKi values. J Pharmacol Exp Ther 1989; 251:238–45 Google Scholar
70 Meltzer HY, Nash JF. Effects of antipsychotic drugs on serotonin receptors. Pharmacol Rev 1991; 43:587–604 Google Scholar
71 Wadenberg M-LG, Browning JL, Young KA, Hicks PB. Antagonism at 5-HT2A receptors potentiates the effect of haloperidol in a conditioned avoidance response task in rats. Pharmacol Biochem Behav 2001; 68:363–70 Crossref, Google Scholar
72 Seeman P, Corbett R, Van Tol HHM. Atypical neuroleptics have low affinity for dopamine D2 receptors or are selective for D4. Neuropsychopharmacology 1997; 16:93–110 and 1997; 16:127–35 Crossref, Google Scholar
73 Seeman P, Kapur S. Olanzapine binding to dopamine receptors in vitro and in vivo. In: Olanzapine (Zyprexa) a novel antipsychotic. Tran PV, Bymaster F, Tye N, Herrera J, Breier A, Tollefson G, Eli Lilly and Co, editors. Philadelphia (PA): Lippincott Williams and Wilkins; 2000. p 3–24 Google Scholar
74 Nyberg S, Eriksson B, Oxenstierna G, Halldin C, Farde L. PET study of D2- and 5-HT2 receptor occupancy induced by risperidone in schizophrenic patients. Am Coll Neuropsychopharmacol 1996; 35:184 Google Scholar
75 Nyberg S, Nakashima Y, Nordström A-L, Halldin C, Farde L. Positron emission to mography of in-vivo binding characteristics of atypical antipsychotic drugs. Review of D2 and 5-HT2 receptor occupancy studies and clinical response. Br J Psychiatry 1996; 168:40–4 Crossref, Google Scholar
76 Trichard C, Paillere-Martinot ML, Attar-Levy D, Recassens C, Monnet F, Martinot JL. Binding of antipsychotic drugs to cortical 5-HT2A receptors: a PET study of chlorpromazine, clozapine, and amisulpride in schizophrenic patients. Am J Psychiatry 1998; 155:505–8 Crossref, Google Scholar
77 Invernizzi RW, Cervo L, Samani R. 8-Hydroxy-2-(Di-N-propylamino) tetralin, a selective serotonin-1A receptor agonist, blocks haloperidol-induced catalepsy by an action on raphe nuclei medianus and dorsalis. Neuropharmacology 1988; 27:515–8 Crossref, Google Scholar
78 Wadenberg M-L. Antagonism by 8-OH-DPAT, but not ritanserin, of catalepsy induced by SCH23390 in the rat. J Neural Transm 1992; 89:4–9 Crossref, Google Scholar
79 Weiner DM, Burstein ES, Nash N, Croston GE, Currier EA, Vanover KE, and others. 5-hydroxytryptamine-2A receptor inverse agonists as antipsychotics. J Pharmacol Exp Ther 2001; 299:268–76 Google Scholar
80 Remington G, Kapur S, Shammi S, Seeman P. “Extended” dosing as an alternative to “intermittent” or “targeted” antipsychotic therapy: Rationale and pilot study. Schizophr Res 2001; 49 (Suppl 1,2):243 Crossref, Google Scholar