Circadian Rhythms in Health and Disease
Abstract
Circadian rhythms refer to the regulation of physiology in a 24-hour cycle and are involved in our sleep-wake cycle, core body temperature, hormone secretion, hunger, mood, and more. Circadian rhythm disorders arise from a misalignment of the timing of our circadian rhythms regulated by the suprachiastmatic nucleus (SCN) and the normal sleep-wake times required to meet the demands of our daily routines. The biological markers of circadian rhythm are melatonin levels and core body temperature, although diagnosing circadian rhythm disorders is done mainly by clinical history, sleep logs, and actigraphy. Delayed sleep phase syndrome, non-24-hour sleep wake disorder, advanced sleep phase syndrome, shift work sleep disorder, and jet lag are the circadian rhythms disorders discussed in this review. Treatments for these including melatonin, light therapy, chronotherapy, and pharmacotherapy are reviewed for each. Additional studies are needed to better determine the optimal timing and dosing for melatonin for circadian rhythm disorders and for insomnia.
Introduction
Circadian rhythms are biological events that repeat roughly every 24 hours. The word circadian is derived from the Latin circa (meaning about) and die (meaning day). These rhythms regulate our sleep-wake cycle, core body temperature, hormone secretion, hunger, and more. They are all controlled by a biological clock in the anterior hypothalamus called the suprachiasmatic nucleus (SCN). The suprachiasmatic nucleus regulates the periodicity of these events to make them occur at the same time every day regardless of outside environmental factors. This biological clock can be entrained by a zeitgeber (German for time-giver) such as the light and dark cycle (1). Light reaches the suprachiasmatic nucleus through melanopsin-containing retinal ganglion cells (2, 3) in our eye whose fibers pass through the retinohypothalmic tract (4).
Circadian rhythms are present in all species and even occur in single celled organisms. It is important to know that not all biologic processes occur in a circadian rhythm: infradian rhythms are those rhythms that occur with a periodicity greater than 24 hours, such as a woman’s menstrual cycle. Ultradian rhythms are those rhythms that occur more frequently than every 24 hours and include the classic example of the sleep cycle with REM sleep occurring approximately every 90 minutes. Nevertheless, the focus on this article will be on the disorders that affect our circadian rhythms.
Possibly the world’s first chronobiologist was Jean-Jacques d’Ortous de Mairan (5). who noted that the leaves of the heliotrope plant, mimosa pudica, would open and close once per day. He keenly observed that these plants would continue to open and close in their natural manner even when the plant was either in simulated sunlight for 24 hours or in constant darkness for the same period of time. He and his colleagues postulated that the control of these rhythms was due to an intrinsic biological rhythm instead of in response to the light-dark cycle.
There are many biological processes that follow a circadian distribution. One well-known phenomenon among primary care physicians is the diurnal variation in serum cortisol levels. These levels peak in the early morning and reach their lowest levels in the early evening, and knowledge of this information is vital when physicians workup adrenal insufficiency. Thyroid-stimulating hormone (TSH) also has a circadian variation with a nadir between 4:00 p.m. and 8:00 p.m. and maximum level between 2:00 a.m. and 4:00 a.m. Subsequently, free T3 and free T4 levels have similar rhythms but are delayed with respect to TSH (6). Core body temperature is yet another example of a circadian rhythm phenomenon and is described below with respect to its importance in its relation to light therapy.
Although it is much harder to measure, circadian rhythms also play an important role in mood disorders. Controlled administration of light and melatonin has been shown to help improve mood in both bipolar disorders and major depression (7–9). Additionally, in bipolar patients a single polymorphism in the human Clock gene was found to influence bipolar characteristics. Those patients who had a thymine to cytosine substitution (3,111T→ 3,111C) were found to have a significant delay in sleep onset (10). Patients with one copy of the 3,111C variant had an increased frequency of insomnia during their depressive episodes and during their lifetime. Furthermore, if a patient had two copies of this allele then they had an even further increased rate of recurrence of their insomnia (11–13).
Circadian Physiology
Currently sleep and wakefulness are held in the balance of a two-process model (Figure 1). Process C is the circadian rhythm, which oscillates in a 24-hour periodicity causing us to sleep and wake up at roughly the same time every day. Process S is our homoestatic sleep drive, where sleep propensity increases with wakefulness and diminishes with sleep (14). Where Process C is controlled by the suprachiasmatic nucleus, it has recently been proposed that Process S and the sleep drive increases with the buildup of adenosine in the basal forebrain which then decreases when we sleep (15). These two processes can become misaligned either voluntarily or though primary disease such as the circadian rhythm disorders.
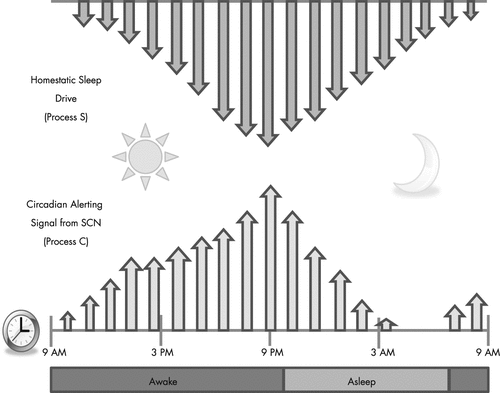
Currently there are two well-established biologic markers of our circadian rhythm: the measurement of melatonin levels and the measurement of core body temperature. The pineal gland secretes melatonin 2 hours before our natural sleep time (16). Because melatonin secretion is suppressed by light (17), in sleep research laboratories melatonin is measured in dim light to evaluate circadian entrainment. The time of this release is referred to as dim light melatonin onset (DLMO) (18). Another marker of a patient’s circadian rhythm is the minimum core body temperature, which reaches its nadir 2–4 hours prior to awakening. Core body temperature minimum marks the singularity of the circadian rhythm, which can be thought of as the pivoting point or fulcrum for light therapy in circadian rhythm disorders. When light is presented before this point, a phase delay is created, and when light is presented after this point, a phase advancement occurs. For example, light occurring in the early evenings before bedtime creates a phase delay, and light in the early morning hours creates a phase advancement (Figure 1).
Melatonin acts in the opposite manner as light does. Melatonin advances the biological clock when given in the evenings (19, 20). There is strong evidence to support that melatonin, if timed correctly, can help advance a patients sleep phase, but one of the major problems with melatonin is that both the optimal dose and timing are debated (21–23). Additionally, potency may also be a variable as the product is not regulated by the Federal Drug Administration (FDA). Despite the fact that it is an endogenous hormone, side effects such as headache, nausea, and feelings of morning grogginess have all been reported.
Assessment and Differential Diagnosis
Circadian rhythm disorders arise from a misalignment of the timing of circadian rhythms and the normal sleep-wake times required to meet the demands of our daily routines. The key to the assessment of circadian rhythm disorders is a detailed history as this will help guide treatment (Table 1). The Diagnostic and Statistical Manual of Mental Disorders (DSM-V) and the International Classification of Sleep Disorders-2 (ICSD-2) are two resources that are available to help clinicians make accurate diagnoses. (The ICSD-3 is expected to be released in mid-2014.) Typically patients with circadian rhythm disorders complain of sleeping at inopportune times, whether it is too late in the evening or too early, and they may complain of either insomnia or excessive daytime sleepiness. In general, an Epworth Sleepiness Scale should be administered for most sleep complaints. It is important to get accurate bedtimes and sleep onset times to determine sleep latency. It is also important to know if the patient reports sleep fragmentation as this is less commonly seen in the primary circadian rhythm disorders. Sleep logs are highly recommended as they can reveal sleep patterns and can aid in the diagnosis. Actigraphy can be used as well and often in combination with sleep logs if there is still clinical uncertainty in the diagnosis.
Common Doses | Uses and Timing | Common Side Effects | Serious Side Effects | |
---|---|---|---|---|
Modafinil(Provigil) | 100 mg, 200mg | SWSD#: 200 mg prior to start of night shift. | Headache, nausea, anxiety, insomnia, palpitations. Induces CYP3A4 liver metabolism and may reduce efficacy of steroidal contraceptives. | Psychosis, mania, Stevens Johnson |
Armodafinil (Nuvigil) | 75 mg, 150 mg, 250 mg | SWSD#: 150 mg prior to start of night shift. Jet lag*: 150 mg Q AM | Same as modafinil | Same as modafinil |
Melatoninb | 0.5–12 mg | DSPS*: 0.5–5 mg, 13–14 hours after natural wake-up time. Non24*: 10 mg QHS 2 hours prior to DLMO for 1 month followed by 1 mg after entrainment. SWSD*: 30 minutes before daytime sleep. East Travel*: 5 mg QHS starting 5 days prior to travel though dose is debated. | Headache, irritability, abdominal cramps, daytime fatigue | None |
Bright Lightb | 10,000 lux | DSPS and Non24*: 1–2 hours after core body temperature minimum.ASPS*: 7:00 p.m.–9:00 p.m. | Headache, eye strain | None |
A physical examination is important to evaluate for other causes of sleep disorders such as obstructive sleep apnea. Polysomnography is not indicated for circadian rhythm diagnosis but may be ordered when other sleep disorders are suspected such as periodic limb movement disorder, narcolepsy, or obstructive sleep apnea syndrome. In the past, circadian rhythm disorders were categorized into two main groups: primary circadian rhythm disorders and secondary circadian rhythm disorders. Primary circadian disorders occur because of an alteration of the timing of the circadian clock such as seen in delayed sleep phase syndrome and advanced sleep phase syndrome. Secondary circadian rhythm disorders occur when the environment in volitionally changed relative to the endogenous circadian rhythm as is seen in jet lag or shift work sleep disorder (SWSD). It is very important to note that DSM-V has removed the categorization of primary and secondary causes of circadian rhythm disorders. It is instead more important for the clinician to list the co-occurring sleep, medical, and psychiatric disorders without assigning causality. Furthermore, jet lag disorder has been removed from the DSM-V altogether. The authors of this article, however, feel it is important to discuss jet lag as it is an important area of interest and much can be learned about circadian rhythms from jet lag and its treatment. Technically speaking, jet lag is the most common of circadian misalignments, but the most common circadian complaint presenting to a sleep disorders clinic is delayed sleep phase syndrome.
The importance of recognizing and appropriately treating these disorders is greater than merely treating the social problems associated with the misalignment of sleep timing. There are chronic medical consequences stemming as a result of these disorders. For example, in a study (24) that only lasted 10 days in a controlled environment, subjects underwent forced dysynchrony and slept at various times during the day and night. After a meal, despite an increase in postprandial insulin levels, glucose levels also increased demonstrating insulin resistance. Researchers also showed a decrease in leptin levels. Leptin is a hormone that normally decreases appetite and increases energy expenditure; low leptin levels contribute to the development of obesity. Researchers also demonstrated an increase in mean arterial pressure levels demonstrating how dysynchrony can lead to hypertension and cardiovascular disease.
Circadian Rhythm Disorders
Delayed Sleep Phase Syndrome
Delayed sleep phase syndrome (DSPS) is characterized by sleep onset insomnia (without sleep maintenance insomnia), that causes excessive daytime sleepiness, specifically with difficulty waking up in the morning to attend to daily obligations such as work or school. The prevalence of DSPS is about 2% of the general population with a tendency to effect young adults and adolescents (25, 26). In delayed sleep phase syndrome there is a high prevalence in both bipolar and unipolar depression. In one study using actigraphy, 62% of bipolar patients had delayed sleep phase when depressed compared with 30% of unipolar depression patients and 10% of healthy controls (27).
DSM-V criteria indicate that the disorder can be classified into three subtypes. Episodic type patients have symptoms that last at least 1 month but less than 3 months. Persistent type patients have symptoms that last 3 months or longer. Recurrent type subtype patients have two or more separate episodes that occur within the space of 1 year. These patients present with insomnia at night and have difficulty waking up in the early morning. It can be further specified if the disorder is familial or overlaps with non-24-hour sleep wake syndrome.
Mutation in the Per3 gene has been implicated in some families with delayed sleep phase syndrome (28). Patients fall asleep anywhere from 1:00 a.m. to 6:00 a.m. and have extreme difficulty waking up in the morning and can sleep as late as the early to midafternoon. Sleep logs or actigraphy can aid in the diagnosis and can be particularly helpful if a patient’s history is internally inconsistent or if the history is not concordant with the patient’s bed partner or parents. Even more objective data can be obtained through the use of salivary melatonin excretion or measurement of core body temperature. Patients with delayed sleep phase syndrome have their DLMO delayed by 2 hours or more and similarly, their core body temperature is delayed by the same amount of time when compared with the normal population (29). Although these objective measures can be obtained, sleep logs are very predictive of these measures and are easier to acquire (30).
Treatment for delayed sleep phase syndrome should be directed at adjusting the circadian rhythm through a combination of behavioral changes, evening melatonin, and bright light therapy. First, the patient should adhere to good sleep hygiene including the avoidance of caffeine in the afternoon and additionally avoid bright light in the evenings, including the light from computer screens, laptops, tablet PCs, and televisions. Melatonin should be given 3–6 hours prior to DLMO. In one study, melatonin doses at 0.3 and 3 mg were given to patients at variable times and compared with placebo to look for optimal dosing and the greatest phase advancement effect. The study found that the dose did not change the effect of the phase advancement, but the study did find that when melatonin was given 6.5 hours prior to DLMO, it produced the greatest phase advancement compared with other timings (31). This timing corresponds to 13–14 hours after natural wakeup time (32). Although the importance of this study was noted in the 2007 AASM practice parameters, and even though melatonin was recommended in the treatment guidelines, the AASM guidelines still indicated that the dose and timing of melatonin administration needed more study.
The importance of light therapy cannot be overstated in the treatment of DSPS. In the morning, bright light therapy in the form of 10,000 lux should be presented to further advance the circadian rhythm and core body temperature. Light will shift the circadian rhythm in a dose-dependent manner (33) (see Figure 2 and Table 1). It should be presented shortly after awakening and care should be taken not to present bright light too early to a patient’s core body temperature minimum as this action could inadvertently delay sleep time even further as demonstrated by the following example.
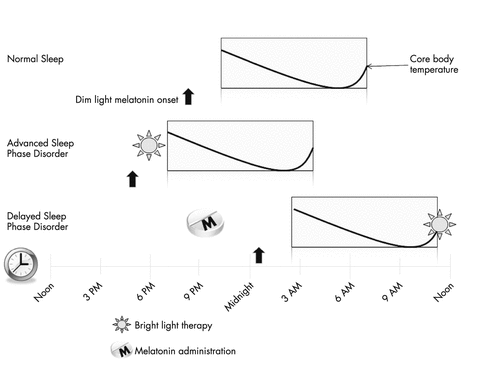
A 16-year old boy who is a sophomore in high school presents with the inability to fall asleep until 3 a.m. and on weekends his preferential wakeup time is 11 a.m. In this example, DLMO is around midnight to 1 a.m., and the patient’s core body temperature minimum may be around 8 a.m. The patient’s parents forcefully wake the patient up every day at 6:30 a.m. with multiple alarms and by dragging him out of bed so the patient can wake up, have breakfast, and get to the bus to make it to school on time. By getting him up early, they expose him to bright sunlight from the outside world before his core body temperature minimum. This action causes light to actually delay the patient’s circadian rhythm even further. Parents may bring their child to a sleep clinic completely surprised that despite the fact their child wakes up early, and is tired or sleepy at school in the morning, he cannot fall asleep at a reasonable hour. They are more stunned to realize they may be the ones contributing to the underlying problem.
A final therapy worth discussing briefly for the treatment of DSPS is chronotherapy. There are no controlled clinical trials looking at its efficacy, but prescribing further delay of the patient’s sleep schedule can help the patient readjust the circadian clock. Since there are no trials, there is no consensus as to how long the therapy should be performed or how much additional sleep delay should be prescribed. For this therapy a patient is asked to stay up a few hours later every few nights until they eventually reach a socially acceptable sleep time. The difficulty in this practice is that patient would need to miss work or school, as they would need to sleep during most of the day until they finally reach a desired sleep time. However, for some patients who are already missing a substantial amount of work or school this therapy may be better than the alternative.
Non-24-Hour Sleep Wake Disorder
Non-24-hour sleep wake disorder is also referred to as “free-running circadian rhythm disorder,” or “non-entrained circadian rhythm disorder.” In this disorder the patient’s circadian rhythm is not entrained by the light and drifts toward the later hours. Classically, this disorder is seen in blind individuals but can occur rarely in sighted individuals. The ratio of men to women is 2.6:1 in both sighted and blind individuals. The disorder occurs because the natural circadian rhythm is not exactly 24 hours, but is thought to be about 24.2 hours (34). In sighted individuals it is postulated that the reason they develop this disorder is because they are less photosensitive than normal individuals (35). In the absence of external queues the patient will fall asleep at later and later times every day. At times they will sleep during socially acceptable sleep times but only by coincidence when their clock is in alignment with social norms.
The clinical diagnosis is made primarily through careful history and sleep logs. The patient will complain of periods of insomnia during the evenings and hypersomnia during the day. This disorder greatly impacts a patient’s ability to work, perform in school, or even participate in social activities. Actigraphy can be very helpful as it shows a classical rightward shift of sleep-wake times.
For blind individuals the only treatment available until recently was melatonin. In sighted individuals the treatment is similar to delayed sleep phase syndrome with careful timing of light and melatonin. Patients must also be vigilant to try to maintain behavioral queues as well such as planned activities, timing of meals, etc. One recommended approach to melatonin treatment for free-running circadian rhythm disorders is to start with high dose melatonin (3 mg−10 mg) 2 hours prior to predicted DLMO nightly for 1 month. Once entrainment occurs over a period of 3–9 weeks, regular low dose melatonin should be continued to prevent a relapse (36). With clinical trials now completed (37), the drug Tasimelteon was recently approved by the FDA for the treatment of non-24-hour sleep wake disorder in the blind. Tasimelteon is thought to reset the master body clock in the SCN to synchronize the body’s melatonin and cortisol circadian rhythms with the day-night cycle.
Advanced Sleep Phase Syndrome
Advanced sleep phase syndrome (ASPS) is characterized by the inability to stay up in the evenings with early bedtimes and early morning awakenings. A patient’s chief complaint is the inability to stay awake and hypersomnolence in the early evening along with early morning awakenings. The onset is during middle age and some studies suggest that age is a risk factor for ASPS as circadian pacemaker phase advances with aging (38). Two gene mutations identified in families with ASPS are Per2 and casein kinase 1, delta gene (26, 43), and when making a DSM-V diagnosis it should be indicated if there is a family history of this disorder. Patients are generally physically unable to stay up for social gatherings in the evening. APSP is less common than DSPS but the prevalence is likely underreported as many patients with this disorder tend to find jobs that allow them to maintain this lifestyle and do not seek treatment for it. In fact, many people with advanced sleep phase syndrome are rewarded professionally because they often show up very early to work. Patients with this syndrome usually fall asleep between 6 p.m. and 9 p.m. and will thus wake up from 2 a.m. to 5 a.m.
Again, detailed history is important to help distinguish ASPS from early morning awakenings due to depression, as this diagnosis can be made entirely from the sleep history. Sleep diaries and wrist actigraphy can also be helpful to make the diagnosis if more objective data are needed. The Horne-Ostberg questionnaire is also a useful tool to help identify morning-type versus evening-type individuals (40). A polysomnogram is not necessary or indicated for the diagnosis of ASPS but it if it is to be done to evaluate for other sleep disorders, then care should be taken to perform the polysomnogram during the patient’s normal sleep time because early onset REM sleep may appear and the study may be limited by a low total sleep time.
American Academy of Sleep Medicine (AASM) guidelines indicate that evening bright light exposure is the only current treatment available for ASPS (Figure 2). Theoretically, morning melatonin would help to delay an ASPS patient’s circadian rhythm, and although the AASM recommends evening melatonin for DSPS, they believe there is insufficient data to assess the safety and efficacy of timed morning melatonin administration for ASPS.
Shift Work Sleep Disorder
According to the Bureau of Labor Statistics, approximately 18% of the workforce is currently participating in shift work (41). The prevalence of shift work disorder (SWSD) is estimated to be about 10% in shift workers (42) but as high as 40% in nurses (43). The ICSD-2 states that shift workers meet the following criteria: patients must (i) complain of insomnia or excessive sleepiness temporally associated with a recurring work schedule in which work hours overlap with the usual time for sleep; (ii) symptoms must be associated with the shift work schedule over the course of at least one month; (iii) sleep log or actigraphy monitoring for ≥7 days demonstrates circadian and sleep-time misalignment; and (iv) sleep disturbance is not better explained by another sleep disorder, mental disorder, a medical or neurological disorder, medication use, or substance use disorder. In a similar manner as DSPS, this disorder can also be subtyped as episodic, persistent, or recurrent by the same criteria.
Other symptoms of SWSD include chronic fatigue, malaise, mood disorders, decreased libido, dyspepsia, and other nonspecific complaints (38). Shift work, even without shift work disorder, has also been linked to many other disorders, including an increased risk of breast cancer (44), diabetes (45), hypertension (26), and depression (46). In patients with shift work disorder, patients have an increased frequency of drowsy driving and an increased risk of work-related injury that is directly related to the amount of shifts worked (44, 47).
The overnight shift causes the most severe symptoms but patients who work any shifts may also experience symptoms if the shift causes them to sleep at times that are misaligned with their endogenous circadian rhythm. Several factors may increase susceptibility to SWSD, including aging, presence of other sleep-related disorders, and the extent of daytime activities and commitments. On days when patients are not working their routine shift, attempts to sleep at socially normal times further worsen the misalignment of the circadian clock.
As with all sleep disorders, treatment first begins with creating good sleep habits, such as avoiding caffeine prior to scheduled sleep time, sleeping in a dark room, and maintaining routine sleep and wake times if possible. Proper nap timing before the beginning of an overnight shift has also been shown to be helpful in reducing symptoms of excessive daytime sleepiness (48). When an overnight shift worker leaves his or her work to go home, avoiding bright light exposure by wearing sunglasses helps prevent circadian misalignment. Additionally, the use of modafinil or armodafinil has been shown to reduce symptoms of excessive daytime sleepiness during work (49). A dose of 150 mg of armodafinil or 200 mg of modafinil has been shown to improve wakefulness when administered prior to the start of a shift (Table 1) (50). There have been multiple studies on the effects of melatonin. These studies show that sleep quality improves but melatonin does not help evening alertness (51–53). AASM guidelines indicate that the effectiveness of sleep quality has not been dose-responsive and some studies showed efficacy in as little as 1.8–3 mg of timed melatonin (21). These guidelines also indicate that sedative hypnotics can be used to promote sleep during the day but carryover effects need to be addressed so the patient is not sleepy when scheduled to commute to work.
Jet Lag Disorder
Jet lag disorder is the most temporary of the circadian rhythm disorders. Although not every traveler will develop jet lag, most will experience some symptoms. It is a result of the timing of the circadian clock becoming misaligned from the timing of wake and sleep required in the new environment. Symptoms generally occur 1–2 days after travel and include complaints such as insomnia, excessive daytime sleepiness, fatigue, malaise, irritability, GI upset, and other nonspecific symptoms. Symptoms are dependent to the number of time zones changed. Westward travel is easier to adapt to than eastward travel because westward travel is corrected by delaying sleep onset (thus creating a greater homeostatic sleep drive). Eastward travel requires the traveler to advance their sleep time or attempt to fall asleep when their sleep drive is low. Older adults have a harder time adapting to jet lag than younger individuals (54, 55).
A nonpharmacological approach that may minimize the effects of jet lag is timed light exposure and altering rest/activity times, although this approach has not been well evaluated. Following eastward flight, exposure to the local light-dark cycle usually accelerates adaptation after jet travel between 2 to 10 time zones. Additionally, shifting sleep/wake schedules earlier by 1 hour per day for 3 days prior to eastward travel may reduce jet lag symptoms. Westward travel is considered to be easier to adjust to than eastward travel; in anticipation of travel, travelers may delay their sleep/wake schedules in order to accelerate adjustment following arrival in the new time zone. For trips that are 2 days or fewer in either direction, advance preparation may not be necessary.
Currently, there are no approved pharmacologic agents for the treatment of jet lag. In a phase 3, double blind, randomized placebo-controlled clinical trial, armodafinil, at a dose of 150 mg, improved alertness demonstrated by mean sleep latency on a multiple sleep latency test and demonstrated improvement in jet lag symptoms through patient surveys (Table 1) (55). However, the FDA has not approved the use of this agent for the treatment of jet lag, and this study was performed after the AASM made its recommendations on the treatment of jet lag disorder. Melatonin has been studied extensively for the treatment of jet lag disorder. Many studies show melatonin is effective to reduce jet lag symptoms when taken prior to bedtime for eastward travel, but the dose is still debated (21, 57). Two studies demonstrated melatonin’s efficacy when traveling westward for 12 time zones or more (58, 59).
Future Directions
The field of sleep medicine is rapidly expanding and there are still many areas where research is needed. As in many other fields of medicine, genetic testing may one day play a role in the diagnosis and treatment of these circadian rhythm disorders. Tests for mutations in Per genes (period homolog genes) and other Clock genes may allow clinicians to create more focused treatment plans. Additional studies are needed to better determine the optimal timing and dosing for melatonin for circadian rhythm disorders and for insomnia. New MRI technology is emerging called Diffusion Tensor Imaging, which can identify a patient’s preferred chronotype by analyzing cortical white matter tracts in a noninvasive manner (60). It will be interesting to see how this technology affects the field and perhaps in the future, if it may be able to guide or predict response to treatment.
1 : Physiology of circadian entrainment. Physiol Rev 2010; 90:1063–1102 [Physiology of circadian entrainment.]Crossref, Google Scholar
2 : Regulation of mammalian circadian behavior by non-rod, non-cone, ocular photoreceptors. Science 1999; 284:502–504Crossref, Google Scholar
3 : Role of melanopsin in circadian responses to light. Science 2002; 298:2211–2213Crossref, Google Scholar
4 : Pineal N-acetyltransferase and hydroxyindole-O-methyltransferase: control by the retinohypothalamic tract and the suprachiasmatic nucleus. Brain Res 1979; 174:245–262Crossref, Google Scholar
5 : Circadian clocks: what makes them tick? Chronobiol Int 2000; 17:433–451Crossref, Google Scholar
6 : Free triiodothyronine has a distinct circadian rhythm that is delayed but parallels thyrotropin levels. J Clin Endocrinol Metab 2008; 93:2300–2306Crossref, Google Scholar
7 : Light therapy for seasonal and nonseasonal depression: efficacy, protocol, safety, and side effects. CNS Spectr 2005; 10:647–663, quiz 672Crossref, Google Scholar
8 : Melatonin, circadian rhythms, and the clock genes in bipolar disorder. Curr Psychiatry Rep 2009; 11:488–493Crossref, Google Scholar
9 : Manipulating melatonin in managing mood. Acta Psychiatr Scand Suppl 2013; (444):16–23Crossref, Google Scholar
10 : Actimetric evidence that CLOCK 3111 T/C SNP influences depressive insomnia and activity patterns in bipolar depression. Am J Med Genet B Neuropsychiatr Genet 2007; 144B:631–635Crossref, Google Scholar
11 : Genetic dissection of psychopathological symptoms: insomnia in mood disorders and CLOCK gene polymorphism. Am J Med Genet B Neuropsychiatr Genet 2003; 121B:35–38Crossref, Google Scholar
12 : Insomnia improvement during antidepressant treatment and CLOCK gene polymorphism. Am J Med Genet B Neuropsychiatr Genet 2005; 137B:36–39Crossref, Google Scholar
13 : Influence of CLOCK gene polymorphism on circadian mood fluctuation and illness recurrence in bipolar depression. Am J Med Genet B Neuropsychiatr Genet 2003; 123B:23–26Crossref, Google Scholar
14 Borbely AA, Tobler I. Homeostatic and circadian principals in sleep regulation in the rat. In: Brain Mechanisms of Sleep, edited by McGinty D, editor. New York, NY: Raven, 1985.Google Scholar
15 : Decoupling of sleepiness from sleep time and intensity during chronic sleep restriction: evidence for a role of the adenosine system. Sleep 2012; 35:861–869Crossref, Google Scholar
16 : The innervation of the mammalian pineal gland. Prog Reprod Biol 1978; 4:1–29Google Scholar
17 : Light suppresses melatonin secretion in humans. Science 1980; 210:1267–1269Crossref, Google Scholar
18 : The endogenous melatonin profile as a marker for circadian phase position. J Biol Rhythms 1999; 14:227–236Crossref, Google Scholar
19 : Delayed sleep phase syndrome response to melatonin. Lancet 1991; 337:1121–1124Crossref, Google Scholar
20 : Evaluating the role of melatonin in the long-term treatment of delayed sleep phase syndrome (DSPS). Chronobiol Int 1998; 15:181–190Crossref, Google Scholar
21 . Practice parameters for the clinical evaluation and treatment of circadian rhythm sleep disorders. Sleep 2007; 30:1445–1459Crossref, Google Scholar
22 : A randomized, double-blind, placebo-controlled crossover study of the effect of exogenous melatonin on delayed sleep phase syndrome. Psychosom Med 2001; 63:40–48Crossref, Google Scholar
23 : Melatonin shifts human circadian rhythms according to a phase-response curve. Chronobiol Int 1992; 9:380–392Crossref, Google Scholar
24 : Adverse metabolic and cardiovascular consequences of circadian misalignment. Proc Natl Acad Sci USA 2009; 106:4453–4458Crossref, Google Scholar
25 : The prevalence of delayed and advanced sleep phase syndromes. J Sleep Res 1993; 2:51–55Crossref, Google Scholar
26 : Prevalence of delayed sleep phase syndrome among adolescents. Sleep Res 1988; 17:392Google Scholar
27 : Delayed sleep phase in young people with unipolar or bipolar affective disorders. J Affect Disord 2013; 145:260–263Crossref, Google Scholar
28 : The genetics of mammalian circadian order and disorder: implications for physiology and disease. Nat Rev Genet 2008; 9:764–775Crossref, Google Scholar
29 : Sleep timing and circadian phase in delayed sleep phase. J Biol Rhythms 2009; 24:313–321Google Scholar
30 : Sleep logs of young adults with self-selected sleep times predict the dim light melatonin onset. Chronobiol Int 2002; 19:695–707Crossref, Google Scholar
31 . Phase dependent treatment of delayed sleep phase syndrome with melatonin. Sleep 2005; ;28:1271–1278.Google Scholar
32 : Estimating dim light melatonin onset (DLMO) phase in adolescents using summer or school-year sleep/wake schedules. Sleep 2006; 29:1632–1641Crossref, Google Scholar
33 : Dose-response relationships for setting of human circadian clock by light. Nature 1996; 379:540–542Crossref, Google Scholar
34 : Circadian rhythm sleep disorders. Clin Chest Med 2010; 31:319–325Crossref, Google Scholar
35 : Non-24-hour sleep-wake syndrome in sighted and blind patients. Sleep Med Clin 2009; 4:195–211Crossref, Google Scholar
36 : Circadian rhythm abnormalities. Continuum (Minneap Minn) 2013; 19:132–147Google Scholar
37 EB. Melatonin agonist tasimelteon (VEC-162) for transient insomnia after sleep-time shift: two randomised controlled multicentre trials. Lancet 2009; 373:433–516Crossref, Google Scholar
38 : Therapeutics for circadian rhythm sleep disorders. Sleep Med Clin 2010; 5:701–715Crossref, Google Scholar
39 : Functional consequences of a CKIdelta mutation causing familial advanced sleep phase syndrome. Nature 2005; 434:640–644Crossref, Google Scholar
40 : A self-assessment questionnaire to determine morningness-eveningness in human circadian rhythms. Int J Chronobiol 1976; 4:97–110Google Scholar
41 : A time to work: recent trends in shift work and flexible schedules. Bureau of Labor Statistics. Mon Labor Rev 2007; 130:3Google Scholar
42 : Shift work disorder: overview and diagnosis. J Clin Psychiatry 2012; 73:e09Crossref, Google Scholar
43 : Shift work disorder in nurses—assessment, prevalence and related health problems. PLoS ONE 2012; 7:e33981Crossref, Google Scholar
44 : Light-at-night, circadian disruption and breast cancer: assessment of existing evidence. Int J Epidemiol 2009; 38:963–970Crossref, Google Scholar
45 : The effects of shift work on sleeping quality, hypertension and diabetes in retired workers. PLoS ONE 2013; 8:e71107Crossref, Google Scholar
46 : Shift work sleep disorder: prevalence and consequences beyond that of symptomatic day workers. Sleep 2004; 27:1453–1462Crossref, Google Scholar
47 : Shift work, safety and productivity. Occup Med (Lond) 2003; 53:95–101Crossref, Google Scholar
48 : The impact of a nap opportunity during the night shift on the performance and alertness of 12-h shift workers. J Sleep Res 2002; 11:219–227Google Scholar
49 . Modafinil for excessive sleepiness associated with shift-work sleep disorder. N Engl J Med 2005; 353:478–486Google Scholar
50 Armodafinil for treatment of excessive sleepiness associated with shift work disorder: a randomized controlled study. Mayo Clin Proc 2009; 84:958–972Google Scholar
51 : Melatonin phase shifts human circadian rhythms in a placebo-controlled simulated night-work study. Am J Physiol Regul Integr Comp Physiol 2002; 282:R454–R463Crossref, Google Scholar
52 : Effects of melatonin administration on daytime sleep after simulated night shift work. J Sleep Res 2001; 10:181–192Crossref, Google Scholar
53 : Melatonin as a chronobiotic: treatment of circadian desynchrony in night workers and the blind. J Biol Rhythms 1997; 12:595–603Crossref, Google Scholar
54 : Aging human circadian rhythms: conventional wisdom may not always be right. J Biol Rhythms 2005; 20:366–374Google Scholar
55 : Age-related differences in recovery from simulated jet lag. Sleep 1992; 15:28–40Crossref, Google Scholar
56 : A phase 3, double-blind, randomized, placebo-controlled study of armodafinil for excessive sleepiness associated with jet lag disorder. Mayo Clin Proc 2010; 85:630–638Crossref, Google Scholar
57 : Melatonin for the prevention and treatment of jet lag. Cochrane Database Syst Rev 2002; 2:CD001520Google Scholar
58 : Effect of melatonin on jet lag after long haul flights. BMJ 1989; 298:705–707Crossref, Google Scholar
59 : A double-blind trial of melatonin as a treatment for jet lag in international cabin crew. Biol Psychiatry 1993; 33:526–530Crossref, Google Scholar
60 “Early to bed, early to rise”: diffusion tensor imaging identifies chronotype-specificity. Neuroimage 2014 84:428.Google Scholar