Systematic Review: Autism Spectrum Disorder and the Gut Microbiota
Abstract
Objective:
Autism spectrum disorders (ASD) are a varying group of disorders characterized by deficiency in social interaction and restrictive patterns of behavior and interests. While there are several studies focusing on the neuro-psychiatric pathogenesis of ASD, its etiology remains unclear. The role of gut-brain-axis in ASD has been studied increasingly and a correlation between symptoms and the composition of gut microbiota has been documented in various works. Despite this, the significance of individual microbes and their function is still widely unknown. This work aims to elucidate the current knowledge of the interrelations between ASD and the gut microbiota in children based on scientific evidence.
Methods:
This is a systematic review done by a literature search focusing on the main findings concerning the gut microbiota composition, interventions targeting the gut microbiota, and possible mechanisms explaining the results in children aged between 2 and 18 years of age.
Results:
Most studies in this review found significant differences between microbial communities, while there was notable variation in results regarding diversity indices or taxonomic level abundance. The most consistent results regarding taxa differences in ASD children’s gut microbiota were higher levels of Proteobacteria, Actinobacteria and Sutterella compared to controls.
Conclusion:
These results show that the gut microbiota of children with ASD is altered compared to one of neurotypically developed children. More research is needed to discover whether some of these features could be used as potential biomarkers for ASD and how the gut microbiota could be targeted in therapeutical interventions.
Appeared originally in Acta Psychiatr Scand 2023;148:242–254
Introduction
Autism spectrum disorders (ASD) are a varying group of disorders characterized by deficiency in social interaction and restrictive patterns of behavior and interests. Although varying severity of symptoms and clinical heterogeneity is a hallmark of ASD, most people with ASD face a number of challenges in everyday life. The neuropsychiatric pathogenesis of ASD has been studied extensively. However, its etiology remains unclear.1 No reliable biomarkers for ASD have been established, and the diagnosis is done based on typical behavioral symptoms and neurocognitive performance.2 ASD is primarily treated and rehabilitated with psychosocial methods, while pharmacological treatments can be used to treat impairing behavioral symptoms.3
A significant number of children with ASD have gastrointestinal symptoms alongside with restricted diets.4–6 It is commonly suggested that the gut microbiota has a role in the development of not only somatic, but also psychiatric disorders. The role of gut-brain-axis in ASD has been studied increasingly and a correlation between symptoms and the composition of gut microbiota has been documented in various works. Despite this, the significance of individual microbes and their function is still widely unknown.7
This work aims to elucidate the current knowledge of the interrelations between ASD and the gut microbiota in children based on scientific evidence. This is a systematic review done by a literature search from PubMed database focusing on the main findings concerning the gut microbiota composition, interventions targeting the gut microbiota, and possible mechanisms explaining the results in children aged between 2 and 18 years of age.
METHODS
Search Strategy
A systematic search was conducted in April 2022 using PubMed, using a search filter for the studies written in English. The search terms were: (“autism spectrum disorder” OR ASD OR autistic* OR autism*) AND (microbiota* OR microbiome* OR microflora*) AND (child* OR children*). In all, 383 articles from the years 1998–2022 were found.
Selection Citeria
Studies with non-human subjects as well as reviews, case reports and duplicate works were excluded. Additionally, studies that failed to provide data for the subjects’ microbiota or age and studies with less than 20 participants with ASD were excluded.
BOX 1. Summations
The results show that the gut microbiota of children with ASD is altered compared to one of neurotypically developed children.
Gut microbiota appears to be a contributive factor in especially gastrointestinal, but also behavioral symptoms that are typical for ASD.
It seems that there are certain microbial groups, such as Proteobacteria and Sutterella, and that are robust microbial-related characteristic for ASD.
Limitations
This review is only descriptive and narrative, and there are more advanced statistical methods available, such as large meta-analyses.
The inclusion of a wider range of databases than what was used in this review could provide more knowledge on the subject.
The inclusion criteria for the review were observational prospective and retrospective studies, case–control studies, randomized controlled trials (RCTs) or cohort studies investigating gut bacteria in children aged 2–18 years diagnosed with autism or ASD. The included articles had to include information about the sample size and gut microbiota. The articles had to be written in English. The detection of gut microbiota was to be done by fecal samples and/or gut biopsy. Finally, the analysis of the microbiota was to be done with metagenomic sequencing, 16S rRNA sequencing, quantitative real-time PCR techniques (qPCR), polymerase chain reaction (PCR) or fluorescence in situ hybridization (FISH).
One person went through the material without blinding. However, consensus meetings on article inclusion and exclusion were performed in a group formed additionally by one post-doctoral and one senior researcher. Information about the articles was collected in a spreadsheet, including the publishing year, study design, number of ASD participants and controls, age, sex distribution, ASD diagnosis criteria, the method of collecting gut microbiota samples, sequencing method, and if reported, gastrointestinal (GI) symptoms, diet and antibiotics were acknowledged.
In another spreadsheet, the results were collected. This was done by recording all the significant differences in microbe taxa between study groups and if there was an intervention included. It was also recorded if the study had results concerning alpha index, that is, intra-individual diversity, or beta index, that is, overall inter-individual differences. As the taxonomic systems varied in different works when reporting results, all the results were recorded in the same taxonomic rank and spelling the original work had used. There were no synonyms that needed to be combined. Results were considered comparable only if they were reported in the same taxonomic rank. Studies indicating similar results were compared in terms of confounding factors.
Results
Study Selection
Out of the 383 search results, 72 articles were chosen. Studies unrelated to the topic, studies not written in English, reviews, case reports and duplicate publication were excluded after title and abstract evaluation. Out of those 72 articles further 16 were excluded, because they did not use the specified sequencing methods, did not meet the age or diagnosis criteria, or did not provide sufficient data for the microbiota. Lastly, 5 more studies were excluded due to having less than 20 participants. Finally, there were 51 eligible articles included in this review (Figure 1).8–58
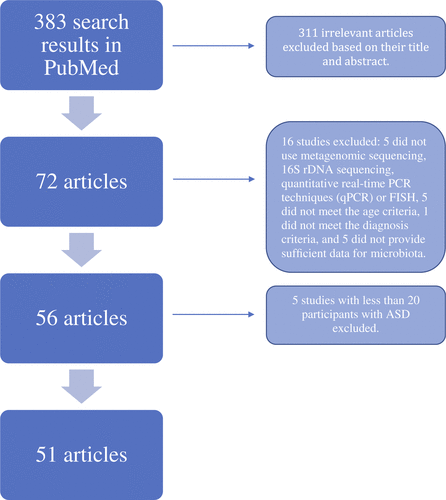
FIGURE 1. Flow chart of study selection
[Publisher’s Note: A color version of the figure, as originally published, appears in the online version of this article at focus.psychiatryonline.org.]
Study Characteristics
Out of the 51 chosen articles the total of 45 were case–control studies, three were randomized controlled trials (RCTs), two were non-randomized controlled trials and one was a cross sectional study without a control group. All but two had a control group, and five studies included an intervention. Articles were published between the years 2005 and 2022. The study subject number ranged from 30 to 286 of which the number of subjects with ASD ranged from 20 to 143. The age of the participants with ASD ranged from 2 to 18 years. Three of the 51 studies included only boys, 44 both girls and boys and 4 did not report the sex distribution of subjects. For ASD diagnosis, The Diagnostic and Statistical Manual of Mental Disorders, Fifth Edition (DSM-5), The Autism Diagnostic Interview-Revised (ADI-R), The Diagnostic and Statistical Manual of Mental Disorders, Fourth Edition DSM-4, International Classification of Diseases 10th Revision (ICD-10), The Autism Treatment Evaluation Checklist (ATEC), The Pervasive Developmental Disorder Behavior Inventory (PDD-BI), The Childhood Autism Rating Scale (CARS), The Griffiths Mental Development Scale (GMDS), The Autism behavior checklist (ABC), The Social Responsiveness Scale (SRS), The Autism Diagnostic Observation Schedule-2 (ADOS-2) and The Pervasive Developmental Disorder Behavior Inventory Screening Version (PDDBI-SV) were used. In nearly every study ASD evaluation was done by a clinician. Five studies did not provide information about the diagnosis criteria for ASD.
For the gut microbiota characterization, 49 studies used fecal samples, one study duodenal biopsy and one both ileal and cecal biopsies. For microbiota sequencing, 37 of the studies used 16S rRNA sequencing, four quantitative real-time PCR, two Clostridium perfringens targeted PCR, one C. Difficile-targeted 16S rRNA, five metagenomic sequencing, and two FISH.
For confounders, 42 of the 51 studies took study subjects' GI-symptoms into account, of which 10 used a GI severity index (GSI or GSI-6), two used GIS (Gastrointestinal Symptom Profile) and one used a GIF (GI failure) score to evaluate the severity of the symptoms. In four studies, subjects with any GI symptoms were not included at all. Thirty five studies acknowledged subjects' diets, and in six of those studies subjects with restrictive diets were excluded. In 40 studies, possible use of antibiotics was considered, of which the total of 38 studies reported that the subjects had not had antibiotics in the last 1–3 months. Study characteristics, such as age, sex distribution, acknowledged diets or comorbidities did not seem to fully explain different results in microbiota studies, when studies with significant group differences regarding alpha diversity, beta diversity or taxa abundances were descriptively compared to studies with no significant findings.
Alpha and beta diversity
Thirty nine studies of all 51 studies analyzed the alpha diversity, that is, intra-individual diversity, of subjects’ gut microbiota. Nineteen studies found no significant difference between study groups. In eight studies, there was higher gut microbiota diversity in the ASD group, while another seven studies found opposite results indicating that children with ASD had in fact lower diversity. Niu et al. found that children with ASD had a higher intestinal microbiota Shannon index, which accounts for richness and evenness of taxa, and a lower Simpson index, which accounts for taxa dominance.25 Two studies had controversial results, and in three studies alpha index seemed to be affected by age or ASD-related symptoms.
Thirty studies analyzed the gut microbiota for overall inter-individual differences in microbiota composition, that is, beta diversity, of which 26 confirmed that ASD children have a significantly different gut microbiota composition compared to a control group and two studies found no significant difference between study groups. Tomova et al. found that dietary habits accounted for the difference in the beta diversity of ASD children, while Fouiquier et al. discovered that beta diversity was affected by geographical location when comparing two ASD groups that lived in different locations (Table 1).38,46
| Result | Number of studies | References |
---|---|---|---|
α-diversity | ASD > control group | 8 | 11–13,27,33,39,42,58 |
| ASD < control group | 7 | 15,17,18,20,29,32,54 |
| No significant group difference | 19 | 8–10,16,19,21,22,26,30,31,35,38,45,47,49–51,56 |
β-diversity | Gut microbiota composition significantly different between ASD and control groups | 26 | 9–13,15–17,19–22,25–27,29–33,40,47,49–51,58 |
| No significant group difference | 2 | 54,56 |
TABLE 1. Alpha and beta diversities in autism spectrum disorder (ASD) participants compared to controls
Specific Phyla and Genus Level Differences
In phylum level, Proteobacteria was found to be increased in autistic samples in five studies.8,9,12,40,56 In contrast, Zou et al. found that Proteobacteria was decreased in children with ASD.39 Actinobacteria was found to be increased in autistic individuals in four studies,8,40,47,56 while Ding et al. reported opposite results.51 In five studies,9,25,30,40 Firmicutes/Bacteroidetes ratio was increased in autistic children’s samples compared to controls. However, Zhang et al., Ahmed et al. and Zou et al. found that the same ratio was in fact decreased in children with ASD.16,35,39
Four studies reported higher levels of Sutterella in autistic samples.11,16,23,43 Another four studies19,30,33,48 found that Lactobacillus was more abundant in the samples of autistic subjects. Enterobacteriaceae levels were noted to be increased in children with ASD in four studies,12,48,50,56 whereas Zou et al. found those levels to be decreased.39 Consistently, Enterobacteriales were found to be increased in autistic samples.12,42
Meanwhile Bacilli,8,10Phyllobacterium48,50 and Oscillospira11,45 were reported more abundant and Coprococcus,20,54Flavonifractor17,39 and Roseburia25,48 less abundant in children with ASD compared to controls.
Liu et al. noted that while the gut microbiota profiles of children with ASD and their mothers had a clear correlation, Clostridium was one of the unique biomarkers found mainly in the ASD children’s samples.29 Consistently, two studies29,58 found that Clostridium genus was more increased in autistic subjects’ samples, while especially C. perfringens was increased in three.34,36,37 Furthermore, in an intervention study by Wang et al. Clostridium levels were decreased after supplementation treatment (Table 2).27
Taxonomic rank | Taxa | Abundance in ASD participants compared to controls | Number of studies | References |
---|---|---|---|---|
Phylym | Firmicutes/Bacteroidetes Ratio | ↑ | 5 | 9,25,30,40,47 |
| | ↓ | 3 | 16,35,39 |
Phylym | Firmicutes | ↑ | 2 | 40,51 |
| | ↓ | 2 | 13,39,56 |
Class | Bacilli | ↑ | 2 | 8,10 |
Genus | Lactobacillus | ↑ | 4 | 19,30,33,48 |
| | ↑ after intervention | 1 | 14 |
Family | Lachnospiraceae | ↑ | 1 | 42 |
| | ↓ | 3 | 29,43,56 |
| | ↑ after intervention | 1 | 10 |
Genus | Coprococcus | ↓ | 2 | 20,54 |
Genus | Roseburia | ↓ | 2 | 25,48 |
Genus | Clostridium | ↑ | 2 | 13,58 |
| | ↓ after intervention | 1 | 27 |
Species | Clostridium perfringens | ↑ | 3 | 34,36,37 |
Genus | Faecalibacterium | ↑ | 2 | 51,54 |
| | ↓ | 3 | 13,42,58 |
Genus | Oscillospira | ↑ | 2 | 11,45 |
Genus | Ruminococcus | ↑ | 3 | 35,45,51 |
| | ↓ | 3 | 13,25,29 |
| | ↑ after intervention | 1 | 10 |
Genus | Dialister | ↑ | 2 | 40,58 |
| | ↓ | 2 | 30,39 |
Genus | Veillonella | ↑ | 1 | 19 |
| | ↓ | 2 | 16,30 |
Phylym | Bacteroidetes | ↑ | 2 | 13,39 |
| | ↓ | 5 | 25,30,40,43,47 |
Genus | Bacteroides | ↑ | 4 | 11,35,39,54 |
| | ↓ | 4 | 21,25,42,47 |
Genus | Prevotella | ↑ | 3 | 13,39,51 |
| | ↓ | 3 | 19,20,54 |
Genus | Prevotella | ↑ | 3 | 13,39,51 |
| | ↓ | 3 | 19,20,54 |
Phylym | Proteobacteria | ↑ | 5 | 8,9,12,40,56 |
| | ↑ | 1 | 39 |
Order | Enterobacteriales | ↑ | 2 | 12,42 |
Family | Enterobacteriaceae | ↑ | 4 | 12,48,50,56 |
| | ↓ | 1 | 39 |
Genus | Escherichia/Shigella | ↑ | 4 | 40,48,50,56 |
| | ↓ | 1 | 39 |
Species | E. coli | ↓ | 2 | 18,21 |
Genus | Sutterella | ↑ | 4 | 11,16,23,54 |
Genus | Phyllobacterium | ↑ | 2 | 48,50 |
Genus | Flavonifractor | ↓ | 2 | 17,39 |
Phylym | Verrucomicrobia | ↓ | 2 | 13,39 |
Phylym | Actinobacteria | ↑ | 4 | 8,40,47,56 |
| | ↓ | 1 | 51 |
Genus | Bifidobacteria | ↑ | 4 | 8,19,40,47 |
| | ↓ | 4 | 13,25,35,45 |
| | ↑ after intervention | 2 | 10,14 |
Genus | Nitriliruptor | ↑ | 2 | 33,38 |
Genus | Collinsella | ↑ | 3 | 30,48,54 |
| | ↓ | 1 | 42 |
TABLE 2. Phyla and taxa level abundances in participants with autism spectrum disorder (ASD) compared to controls when more than one study had similar significant results concerning the taxa
Intervention Outcomes
Grimaldi et al. conducted a randomized placebo-controlled trial with 26 participants with ASD.10 The participants were divided into two groups based on their dietary habits. The intervention was a 6-week treatment with Bimuno® galactooligosaccharide (B-GOS) prebiotics. Children with restricted diets and B-GOS intervention resulted with significantly higher B. longum abundance together with improvements in sociability. In the same study, the effects of exclusion diets on gut microbiota composition and metabolism in ASD children were assessed. In baseline, Bifidobacterium and Veillonellaceae family were less abundant in children on exclusion diets while Faecalibacterium prausnitzii and Bacteroides were found in higher abundance compared to children with an unrestricted diet. Children with restricted diets also were reported with lower scores in abdominal pain and bowel movement. Participants with probiotics, prebiotics, antibiotics, or another dietary supplement drug intake were excluded. Other possible interventions were not considered in statistical analyses.
Another non-blinded randomized controlled trial by Niu et al. studied the intestinal microbiota and probiotics treatment in children with ASD in China.25 Thirty seven children with ASD received a 4-week probiotic treatment combined to an applied behavior analysis (ABA) training while other 28 ASD children were treated with ABA training only. After the intervention the group with combination treatment including both probiotics and ABA training had a greater decrease in ATEC and GI scores compared to the group who did not receive probiotics.
In a double-blinded placebo-randomized controlled trial by Wang et al., a probiotics and fructo-oligosaccharide intervention was conducted in order to modulate the microbiota-gut-brain axis and to improve ASD symptoms.27 In this study, 26 children with ASD were divided into two groups where the first group received both probiotics and fructo-oligosaccharide (FOS) treatment while the second group received placebo supplementation. All subjects were placed in the same hospital ward where they had received identical diet for the preceding 12 months. The duration of the intervention was 30, 60, and 108 days. After the intervention there was an increase in Bifidobacteriales and B. longum in the probiotics + FOS group, while Clostridium and Ruminococcus abundances were decreased. ATEC scored were decreased after probiotics and FOS treatment of 30–60 days. Also 6-GSI scores assessing gastrointestinal symptoms were reduced after intervention. These differences did not occur in the placebo group.
A prospective, open-label study assessing the role of probiotics with no placebo control in children with ASD was done by Shaabam et al. 30 children with ASD were given probiotics supplementation including Lactobacillus acidophilus, Lactobacillus rhamnosus and Bifidobacteria longum for 3 months.27 This resulted in an increase in Bifidobacteria and Lactobacilli levels as well as improved ATEC scores indicating decreased manifestation of autistic behavior. There was also a decrease in body weight and gastrointestinal symptoms compared to the baseline (Table 2).
Li et al. conducted an open-label study where 40 children with ASD were given fecal microbiota transplantation (FMT) for consecutive 4 weeks either orally or in a colonoscopy.49 The composition of the gut microbiota and gastrointestinal and autistic symptoms were evaluated. While the gut microbiota diversity remained unchanged, both gastrointestinal and autistic symptoms were significantly reduced after the treatment. Gastrointestinal symptoms were measured with the GSRS score, which was significantly decreased after 8 weeks. This included symptoms such as abdominal pain, reflux, indigestion, diarrhea, and constipation. Autistic symptoms were evaluated with both the ABC and the CARS scores and were significantly improved 8 weeks after FMT, but no long-term benefits were assessed in this study.
Functional characteristics
In four studies, lower levels butyrate was associated with ASD. Wang et al. and Liu et al. found lower levels of butyrate in the ASD group,27,29 while in another study there were decreases in the abundance of genes linked to production of butyrate in the ASD metagenomes. Furthermore, Zhang et al. found that butyrate and lactate producers were less abundant in the ASD group compared to controls.16 However, other three studies found no significant difference in fecal butyrate levels. Children with ASD also had lower levels of fecal acetic acid.29,32 Liu et al. found that fecal valeric acid was increased in ASD subjects.19 Meanwhile, Dan et al. noticed that valeric acid was slightly increased in autistic children with constipation compared to those without constipation.40
After the Probiotics + FOS intervention study by Wang et al., they reported elevated levels of short-chain fatty acids (SCFAs), approaching those in the control group.27 ASD children had altered glutamate metabolites in addition to an abundance with microbiota associated with glutamate metabolism. Gut 2-keto-glutaramic acid was validated as a potential biomarker for ASD as there was a significant decline of it in the ASD group.32 While the total amount of SCFAs was found to be higher in children with ASD in a study by Ha et al., acetic acid, propionic acid or butyric acid levels showed no significant differences between groups.47 Interestingly calprotectin, a marker for gut inflammation, was found to correlate with behavioral and GI symptoms in ASD.33 This did not occur in the study by Tonkaz et al. focusing on inflammation markers in ASD.57 It was also noted that increased Clostridium botulinum correlated with altered glutamate metabolites32 and in another study, disaccharidase activity.21
Averina et al. found that there were decreases in the abundance of genes linked to production of butyrate, gamma-aminobutyric acid (GABA) and melatonin in the ASD group.28 Consistently, GABA levels in feces were found to be decreased in ASD group in a study by Kang et al.15 However, Wang et al. found no significant difference in plasma GABA levels when comparing ASD group to controls and in an intervention study by Li et al., GABA and serotonin (5-HT) serum concentrations were decreased after fecal microbiota transplantation.27,49 In a study by Wan et al., the microbiome pathways involved in neurotransmitter biosynthesis seemed to be decreased in children with ASD.58 De Angelis et al. found that ASD children had dysregulated metabolism of Flavone-8-acetic acid (FAA).13 In the study by Kang et al., there were higher isopropanol concentrations in the feces on ASD children and in addition, increased serotonin and decreased homovallinic acid, indicating a dopamine metabolism disruption.15
Discussion
The aim of this study was to perform a systematic review of the current knowledge on ASD and the gut microbiota in children. The results show that the gut microbiota of children with ASD is altered compared to one of neurotypically developed children. Most studies in this review found significant differences between microbial communities, while there was notable variation in results regarding diversity indices or taxonomic level abundance. The most coherent results regarding taxa level differences in ASD children’s gut microbiota compared to controls were in Proteobacteria, Actinobacteria and Sutterella levels, that all seemed to be increased in ASD.
Beta diversity describes the dissimilarity between overall microbial communities. The total of 26 studies in this review reported that the gut microbiome of ASD children was significantly different compared to the control samples, while two studies with no significant difference. Beta diversity was also found to be affected by dietary habits38 and geographical location.46 However, results regarding alpha diversity were more contradictory. Most studies did not specify the alpha diversity index; therefore, it remains unclear whether the difference in the diversity is explained by richness of different species or a dominance in a specific species. Liu et al. made similar remarks indicating that while it seems that the gut microbiota is indeed altered in ASD, the significance of diversity in a single sample remains unclear.59
Apart from three studies, all studies that found no significant difference in the alpha diversity had acknowledged the diet of participants. In many of those studies, participants with restricted diets were excluded or all participants were given a similar diet. Diet is known to directly alter the composition of gut microbiota,60 therefore, homogenous diets often observed in ASD children could result in less intra-individual but more inter-individual variety in gut microbiota as well. Three out of five studies that had results indicating that participants with ASD had higher diversity did not report the diets of participants, while most studies with results indicating that ASD children had lower alpha diversity had reported the diets. Berding and Donovan found no difference in alpha diversity when comparing the gut microbiota of ASD children to healthy controls but found that dietary patterns were associated with specific microbial profiles.31 Children who consumed more vegetables, legumes, nuts and seeds, fruit alongside with refined carbohydrates and starchy vegetables had microbiota profiles characterized by lower abundance if Enterobacteriacae, Lactococcus, Roseburia, Leuconostoc and Ruminococcus, while the gut microbiota of children who consumed less of those foods had higher levels of multiple species, including Barnesiellacaea and Alistipes. More restricted diet could, therefore, result in increased abundance of certain species. However, in the same study, diet and microbiota did not predict social deficit scores. In a recent large autism stool metagenomics study by Yap et al., restricted dietary preferences seemed to result in less diverse gut microbiota and additionally, looser stool consistency.52 They did not find evidence for gut microbiota directly contributing to the etiopathogenesis of ASD. There are also other factors, such as age and type of delivery, that could affect the composition of the gut microbiota.42 Of note, as there was great variety in how restricted diets were defined and assessed in the reviewed studies, heterogeneity of diets is likely to affect results. Another source of uncertainty is how much these different diets are associated with GI problems and altered gut microbiota composition. We noted no clear association between study characteristics and whether differences in alpha diversity were reported. These results show that instead of solely focusing on the microbial differences, more research is needed to understand the connection between diet, gut microbiota, and ASD in children, and furthermore, the factors affecting microbial diversity.
Williams et al., Zhang et al., Zhai et al., and Chen, Shi et al. had aligned results indicating that Sutterella is more abundant in children with ASD.11,16,23,54 Williams et al. discovered that Sutterella could be found in the gut microbiota of more than half of children with ASD, while none of the controls had it. The above-mentioned studies included both subjects with and without GI symptoms. These findings could indicate that Sutterella may be associated with ASD regardless of GI symptoms.
Five studies confirmed that Proteobacteria was found in higher abundance in children with ASD. In only one study Proteobacteria levels were decreased. Li et al. found that also the mothers of children with ASD had a higher abundance of Proteobacteria, which could be explained by dietary habits and other environmental factors but also with congenital factors that alter the gut microbiota of children.12 In a study by Plaza-Diaz et al., participants were divided into mental regression and non-mental regression groups based on their ADI-R score. They found that Proteobacteria were more abundant in ASD children with mental regression compared to those with ASD and no mental regression.8 There is evidence for Proteobacteria being associated with inflammatory processes in the GI tract.61,62 These results could indicate that Proteobacteria, that include many potentia1 pathogens such as Escherichia, Vibrio, Helicobacter, Salmonella and Yersinia could affect not only the gastrointestinal tract but also neuropsychiatric function of children.
While there seems to be some features of gut microbial composition that are typical to children with ASD, different clinical pictures of ASD seem to be characterized by differences in the gut microbiota as well.46,53 Ding et al. reported that the severity of ASD symptoms seems to affect the composition of the gut microbiota.42 More severe phenotype of ASD was characterized with higher levels of Lachnospiraceae and Erysipelotrichaceae and lower levels of Faecalibacterium. The observed dose–response in ASD severity and Lachnospiraceae abundance might indicate a causal relationship. The members of Lachnospiraceae family have yielded mixed associations with pathology, and likewise, the were discrepancy in our review regarding associations between Lachnospiraceae abundances and ASD.63 Although the members of Lachnospiraceae family are known SCFA-producers, the differences in microbial networks and cross-feeding patterns may result in different physiological effects. Additionally, it has been suggested that the severity of ASD symptoms could be connected to specific gut microbial characteristics.46,53 In a study by Hua et al. children with ASD were divided into two groups based on whether they had a comorbid sleep disorder,41 and those who suffered from sleep disorder had also more severe ASD symptoms. It was discovered that the microbial profiles of the two groups were significantly distinct. Children with ASD who also suffered from sleep disorders had higher abundance and richness of gut microbiota. Nevertheless, while some of the clinical features of ASD seem to be associated with the gut microbial composition, a correlation between the two is hard to confirm.35
Comorbid gastrointestinal symptoms could be characterized by a distinct microbial profile as well.20 While some studies had excluded all participant with any GI symptoms, others only included those with GI symptoms to both study and control group. This variation could partially explain the heterogeneity of results, although no clear association with participant's GI symptoms seemed to explain the differences in findings. Most studies had not mentioned if the patients were screened for other neuropsychiatric disorders, which could confound the results. Possible microbial differences can also be explained by behavior typical to ASD, such as restrictive eating patterns and potential therapies.
Four studies reported higher levels of Actinobacteria in children when compared to controls. Only one study reported decreased levels of Actinobacteria. In a recent meta-analysis by Chavira et al., higher levels of Actinobacteria in children with ASD was one of the most coherent results.64 It was speculated whether this could indicate that the gut microbiota of children with ASD is underdeveloped, as Actinobacteria is more abundant in younger children. Three trials that included an intervention had interesting results regarding changes in behavioral symptoms among children with ASD. In the study conducted by Grimaldi et al. children with both restricted diet and B-GOS intervention had higher levels of B. longum alongside with improved ATEC scores indicating improvements in sociability.10 Consistently, Wang et al. discovered that Bifidobacteriales and B. longum were significantly increased after probiotics and fructo-oligosaccharide supplementation.27 Both ATEC and 6-GSI score were decreased indicating improvements in autistic and GI symptoms. Shaabam et al. had similar results: after probiotic supplementation, children with ASD had an increase in Bifidobacteria abundance as well as improvements in ATEC scores.14 Meanwhile, Ahmed et al. discovered that the only difference in the gut microbiota of ASD children compared to their healthy siblings was that the siblings had higher levels of Bifidobacteria, which could act as a protecting factor.35B. longum has been previously connected to reduced stress levels,65 which indicates that it has a significant role in the gut-brain-axis function. While this review shows that the abundance of Bifidobacteria seems to vary in children with ASD (Table 2), Bifidobacteria and especially B. longum could be targeted more specifically in probiotic treatment of ASD children in the future, although as concluded in a systematic review by Ng et al., more research with standardized intervention regimen is needed to study the potential benefits of supplementation treatments.66
Some of the studies had also studied different microbial metabolites. These results give potentially more mechanistic insight to understanding the gut-brain-axis. In the current review, multiple studies discovered that SCFAs were associated with ASD. Studies that had looked at SCFA fecal concentrations, SCFA producing bacteria or genes coding SCFA producing enzymes all confirmed differences in children with ASD compared to controls. Differences in SCFA levels can be explained by abundance of certain SCFA producing bacterial taxa or the amount of carbohydrates in diet. Most studies that had reported SCFA levels had excluded participants with major dietary differences, indicating that there were differences in certain SCFA producing bacterial abundances. Two studies confirmed that there was indeed a connection between SCFA concentrations and levels of certain taxa. However, it should be noted that the methods used to detect functional markers varied between studies and was not considered in this review. Four studies found that lower level of butyrate was associated with ASD. However, other three studies found no significant difference in fecal butyrate levels. Butyrate is a SCFA that has been mostly associated with maintaining gut health but there has been growing interest of the neuromodulative effects of butyrate.67 Butyrate has been associated with attenuating ASD behavior in animal models68 and it was discovered that it could affect genes linked to behavior and cognition via energy metabolism by Rose et al.69 In one study, more severe ASD symptoms were connected to microbial taxa involved in butyrate metabolism. Also, acetic acid levels seemed to be lower in ASD. The results of this review further support the idea of butyrate and other SCFAs playing a role in developing both gastrointestinal and behavioral symptoms in ASD, although the number of studies with fecal metabolomics data in child ASD research is still scarce.
It has been speculated whether specific microbial differences could be used as biomarkers for ASD. While this review confirms that there seems to be multiple differences in the gut microbiota of ASD children compared to healthy controls, the specific species that could be used as biomarkers remain controversial. There is significant variety in how the differences are classified, for example, whether the study has looked at phyla or genus level. Most of the studies included in this review used 16s rRNA sequencing to analyze the composition of the gut microbiota, but there were studies using Clostridium perfringens targeted PCR, quantitative real-time PCR, shotgun metagenomics and FISH methods as well. Thereby, results that have been achieved using different methods cannot be fully compared. Almost all studies had used stool samples to analyze the gut microbiota. Using gut biopsies could provide more information on species that cannot be detected in stool samples. Furthermore, this review focused only on gut microbiota as this domain has been researched in the context of ASD sufficiently to conduct systematic literature review, but there is some research on other human microbiota as well, such as a study by Kong et al. discovering alterations in oral microbiota in ASD.9 To develop a full picture of the connection between microbial changes and ASD, more research on non-gut microbiota could be beneficial.
Many studies in this review had a relatively small sample size, most including less than 100 participants. Additionally, in several studies used data was collected in one clinic or hospital. This review includes studies that have been conducted in different continents, including Asia, Europe, Africa, and Northern America, which can explain contradictory results, as the gut microbiota is likely to be affected by environmental and host genetic factors as well as cultural dietary characteristics. All these factors partly explain the complex results in this review. This review is only descriptive and narrative, and there are more advanced statistical methods available, as it was done in a large meta-analysis by West et al.70 The inclusion of a wider range of databases than what was used in this review could provide more knowledge on the subject. Our findings complement those of the meta-analysis, since many datasets are not openly available in public repositories or shared upon request.70 It could be beneficial to study comparative larger cohorts like this, including participants from different locations and utilize replication data sets. This could bring more epidemiological or population level insight on the subject.
All studies in this review had included more male than female participants, three of the studies including only male participants, which is explained by well-known differences in prevalence of ASD among boys and girls.71 Thereby, it should be noted that the sex-ratio of children with diagnosed ASD affects participant recruitment and furthermore, results of a study, and the results might not be as representative when looking at girls with ASD. Also, the selection of controls varied in different studies. Some of the studies had used typically developed siblings as controls, while in other studies, controls were unrelated, which can influence the interpretation of results since the gut microbiota of neurotypical siblings is more similar to ASD group than to unrelated controls.24,35 Nevertheless, most of the studies using siblings as controls did find significant differences between ASD children and their healthy siblings, and studies with both related and unrelated controls would give better insight in shared environmental and host genetic factors. This review highlights what is already known about the gut microbiota and ASD in children.
Conclusions
As a conclusion, gut microbiota appears to be a contributive factor in especially gastrointestinal, but also behavioral symptoms that are typical for ASD. It also seems that there are certain microbial taxa, such as Proteobacteria and Sutterella, and functional markers, such as lower levels of butyrate, that are robust microbial-related characteristic for ASD. Study characteristics do not seem to explain different results in microbiota studies. Probiotic interventions improve the comorbid GI symptoms, and thus it seems that the ASD-related gut microbiota features are interrelated with GI symptoms. However, results concerning probiotic supplements and clinical implementations should be interpreted with caution since the results remain incoherent and found connections are likely to be bidirectional. Based on this review, no causal relationship between any specific taxa and ASD can be implicated, thus, more evidence is needed to assess any probiotic supplementation. More research is needed to discover whether some of these features could be used as potential biomarkers for ASD and how the gut microbiota could be targeted in therapeutical interventions.
1 . An overview of autism Spectrum disorder, heterogeneity and treatment options. Neurosci Bull. 2017;33(2):183-193. doi:
2 . Autism spectrum disorder. Lancet. 2018;392(10146):508-520. doi:
3 . Autismikirjon häiriöt. Lääkärin käsikirja. Kustannus Oy Duodecim; 2018 http://www.terveysportti.fi/apps/dtk/ltk/article/ykt00814 Google Scholar
4 . Medical comorbidities in autism: challenges to diagnosis and treatment. Neurotherapeutics. 2010;7(3):320-327. doi:
5 Association of maternal report of infant and toddler gastrointestinal symptoms with autism: evidence from a prospective birth cohort. JAMA Psychiatry. 2015;72(5):466-474. doi:
6 . Food selectivity and sensory sensitivity in children with autism spectrum disorders. J Am Diet Assoc. 2010;110(2):238-246. doi:
7 The microbiota-gut-brain Axis. Physiol Rev. 2019;99(4):1877-2013. doi:
8 Autism spectrum disorder (ASD) with and without mental regression is associated with changes in the fecal microbiota. Nutrients. 2019;11(2):337. doi:
9 New and preliminary evidence on altered Oral and gut microbiota in individuals with autism Spectrum disorder (ASD): implications for ASD diagnosis and subtyping based on microbial biomarkers. Nutrients. 2019;11(9):2128. doi:
10 A prebiotic intervention study in children with autism spectrum disorders (ASDs). Microbiome. 2018;6(1):133. doi:
11 . Disturbance of trace element and gut microbiota profiles as indicators of autism spectrum disorder: a pilot study of Chinese children. Environ Res. 2019;171:501-509. doi:
12 Correlation of gut microbiome between ASD children and mothers and potential biomarkers for risk assessment. Genom Proteom Bioinform. 2019;17(1):26-38. doi:
13 Fecal microbiota and metabolome of children with autism and pervasive developmental disorder not otherwise specified. PLoS One. 2013; 8(10):e76993. doi:
14 The role of probiotics in children with autism spectrum disorder: a prospective, open-label study. Nutr Neurosci. 2018;21(9):676-681. doi:
15 Differences in fecal microbial metabolites and microbiota of children with autism spectrum disorders. Anaerobe. 2018;49:121-131. doi:
16 . Analysis of gut microbiota profiles and microbe-disease associations in children with autism spectrum disorders in China. Sci Rep. 2018; 8(1):13981. doi:
17 Altered gut microbiota in Chinese children with autism Spectrum disorders. Front Cell Infect Microbiol. 2019;9:40. doi:
18 Functional analysis of gut microbiota and immunoinflammation in children with autism spectrum disorders. Dig Liver Dis. 2019;51(10):1366-1374. doi:
19 Gut microbial dysbiosis in Indian children with autism spectrum disorders. Microb Ecol. 2018;76(4):1102-1114. doi:
20 Reduced incidence of Prevotella and other fermenters in intestinal microflora of autistic children. PLoS One. 2013;8(7):e68322. doi:
21 . Analysis of the duodenal microbiome in autistic individuals: association with carbohydrate digestion. J Pediatr Gastroenterol Nutr. 2017;64(5):e110-e116. doi:
22 Comparison of fecal microbiota in children with autism spectrum disorders and neurotypical siblings in the Simons simplex collection. PLoS One. 2015;10(10):e0137725. doi:
23 . Application of novel PCR-based methods for detection, quantitation, and phylogenetic characterization of Sutterella species in intestinal biopsy samples from children with autism and gastrointestinal disturbances. mBio. 2012;3(1):e00261-e00211. doi:
24 . Differences between the gut microflora of children with autistic spectrum disorders and that of healthy children. J Med Microbiol. 2005;54(Pt 10):987-991. doi:
25 Characterization of intestinal microbiota and probiotics treatment in children with autism Spectrum disorders in China. Front Neurol. 2019;10:1084. doi:
26 . Dietary patterns impact temporal dynamics of fecal microbiota composition in children with autism spectrum disorder. Front Nutr. 2020;6:193. doi:
27 Probiotics and fructo-oligosaccharide intervention modulate the microbiota-gut brain axis to improve autism spectrum reducing also the hyper-serotonergic state and the dopamine metabolism disorder. Pharmacol Res. 2020;157:104784. doi:
28 . The bacterial neurometabolic signature of the gut microbiota of young children with autism spectrum disorders. J Med Microbiol. 2020;69(4):558-571. doi:
29 Altered gut microbiota and short chain fatty acids in Chinese children with autism spectrum disorder. Sci Rep. 2019;9(1):287. doi:
30 New evidences on the altered gut microbiota in autism spectrum disorders. Microbiome. 2017;5(1):24. doi:
31 . Diet can impact microbiota composition in children with autism spectrum disorder. Front Neurosci. 2018;12:515. doi:
32 Alterations in gut glutamate metabolism associated with changes in gut microbiota composition in children with autism Spectrum disorder. mSystems. 2019;4(1):e00321-18. doi:
33 Specificity of gut microbiota in children with autism spectrum disorder in Slovakia and its correlation with astrocytes activity marker and specific behavioural patterns. Physiol Behav. 2020;214:112745. doi:
34 . Detection of Clostridium perfringens toxin genes in the gut microbiota of autistic children. Anaerobe. 2017;45: 133-137. doi:
35 Study of the gut microbiome profile in children with autism spectrum disorder: a single tertiary hospital experience. J Mol Neurosci. 2020;70(6): 887-896. doi:
36 . Incidence of Clostridium perfringens and its toxin genes in the gut of children with autism spectrum disorder. Anaerobe. 2020;61:102114. doi:
37 Impact of clos-tridium bacteria in children with autism Spectrum disorder and their anthropometric measurements. J Mol Neurosci. 2020; 70(6):897-907. doi:
38 . The influence of food intake specificity in children with autism on gut microbiota. Int J Mol Sci. 2020;21(8):2797. doi:
39 Changes in the gut microbiota of children with autism Spectrum disorder. Autism Res. 2020; 13(9):1614-1625. doi:
40 Altered gut microbial profile is associated with abnormal metabolism activity of autism spectrum disorder. Gut Microbes. 2020;11(5):1246-1267. doi:
41 The gut microbiota and associated metabolites are altered in sleep disorder of children with autism spectrum disorders. Front Psychiatry. 2020;11:855. doi:
42 Gut microbiota changes in patients with autism spectrum disorders. J Psychiatr Res. 2020; 129:149-159. doi:
43 Gut bacteria shared by children and their mothers associate with developmental level and social deficits in autism Spectrum disorder. mSphere. 2020;5(6): e01044-e01020. doi:
44 . Sensory processing and gastrointestinal manifestations in autism spectrum disorders: No relation to Clostridium difficile. J Mol Neurosci. 2021;71(1):153-161. doi:
45 Children with autism and their typically developing siblings differ in amplicon sequence variants and predicted functions of stool-associated microbes. mSystems. 2021;6(2):e00193-e00120. doi:
46 The gut micro-biome in autism: study-site effects and longitudinal analysis of behavior change. mSystems. 2021;6(2):e00848-e00820. doi:
47 Altered gut microbiota in Korean children with autism spectrum disorders. Nutrients. 2021;13(10): 3300. doi:
48 Serum oxytocin level correlates with gut microbiome dysbiosis in children with autism Spectrum disorder. Front Neurosci. 2021;15:721884. doi:
49 Fecal microbiota transplantation relieves gastrointestinal and autism symptoms by improving the gut microbiota in an open-label study [published correction appears in front cell infect microbiol. 2021 Nov 23;11:801376]. Front Cell Infect Microbiol. 2021;11:759435. doi:
50 Microbiome-specific statistical modeling identifies interplay between gastrointestinal microbiome and neurobehavioral outcomes in patients with autism: a case control study. Front Psychiatry. 2021;12:682454. doi:
51 . Imbalance in the gut microbiota of children with autism spectrum disorders. Front Cell Infect Microbiol. 2021;11:572752. doi:
52 Autism-related dietary preferences mediate autism-gut microbiome associations. Cell. 2021;184(24):5916-5931.e17. doi:
53 . Application of clustering method to explore the correlation between dominant flora and the autism spectrum disorder clinical phenotype in Chinese children. Front Neurosci. 2021;15:760779. doi:
54 Gut microbial profile is associated with the severity of social impairment and IQ performance in children with autism Spectrum disorder. Front Psychiatry. 2021;12:789864. doi:
55 . Commercial microbiota test revealed differences in the composition of intestinal microorganisms between children with autism spectrum disorders and neurotypical peers. Sci Rep. 2021;11(1):24274. doi:
56 Alteration of the fecal microbiota in Chinese children with autism spectrum disorder. Autism Res. 2022;15(6):996-1007. doi:
57 . Determinants of leaky gut and gut microbiota differences in children with autism Spectrum disorder and their siblings. J Autism Dev Disord. 2023;53(7):2703-2716. doi:
58 Underdevelopment of the gut micro-biota and bacteria species as non-invasive markers of prediction in children with autism spectrum disorder. Gut. 2022; 71(5):910-918. doi:
59 . Altered composition and function of intestinal microbiota in autism spectrum disorders: a systematic review. Transl Psychiatry. 2019;9(1):43. doi:
60 . Feeding the microbiota-gut-brain axis: diet, microbiome, and neuropsychiatry. Transl Res. 2017;179:223-244. doi:
61 . The gut microbiota and inflammatory bowel disease. Semin Immunopathol. 2015;37(1):47-55. doi:
62 . Proteobacteria: microbial signature of dysbiosis in gut microbiota. Trends Biotechnol. 2015; 33(9):496-503. doi:
63 . The controversial role of human gut Lachnospiraceae. Microorganisms. 2020;8(4):573. doi:
64 . Meta-analysis of the autism gut microbiome identifies factors influencing study discrepancies and machine learning classification. bioRxiv. Preprint posted online March 19, 2022. doi:
65 Bifidobacterium longum 1714 as a translational psychobiotic: modulation of stress, electrophysiology and neurocognition in healthy volunteers. Transl Psychiatry. 2016;6(11):e939. doi:
66 . A systematic review of the role of prebiotics and probiotics in autism spectrum disorders. Medicina (Kaunas). 2019; 55(5):129. doi:
67 . Review article: the role of butyrate on colonic function. Aliment Pharmacol Ther. 2008;27(2):104-119. doi:
68 . Sodium butyrate attenuates social behavior deficits and modifies the transcription of inhibitory/excitatory genes in the frontal cortex of an autism model. Neuropharmacology. 2016;102:136-145. doi:
69 Butyrate enhances mitochondrial function during oxidative stress in cell lines from boys with autism. Transl Psychiatry. 2018;8(1):42. doi:
70 Multi-angle meta-analysis of the gut microbiome in autism spectrum disorder: a step toward understanding patient subgroups. Sci Rep. 2022; 12(1):17034. doi:
71 Autism and Developmental Disabilities Monitoring Network Surveillance Year 2008 Principal Investigators; Centers for Disease Control and Prevention. Prevalence of autism spectrum disorders—autism and developmental disabilities monitoring network, 14 sites, United States, 2008. MMWR Surveill Summ. 2012;61(3):1-19. Google Scholar