Nothing Tastes as Good as Skinny Feels: the Neurobiology of Anorexia Nervosa
Abstract
Individuals with anorexia nervosa (AN) engage in relentless restrictive eating and often become severely emaciated. Because there are no proven treatments, AN has high rates of relapse, chronicity, and death. Those with AN tend to have childhood temperament and personality traits, such as anxiety, obsessions, and perfectionism, which may reflect neurobiological risk factors for developing AN. Restricted eating may be a means of reducing negative mood caused by skewed interactions between serotonin aversive or inhibitory and dopamine reward systems. Brain imaging studies suggest that altered eating is a consequence of dysregulated reward and/or awareness of homeostatic needs, perhaps related to enhanced executive ability to inhibit incentive motivational drives. An understanding of the neurobiology of this disorder is likely to be important for developing more effective treatments.
(Reprinted with permission from the Trends in Neurosciences 2013; 36:110–120)
Introduction
Anorexia nervosa (AN) is a disorder of unknown etiology that tends to affect young women [1]. This illness is characterized by restricted eating, severe emaciation, and distorted body image, as well as high rates of chronicity, morbidity, and mortality [1]. It has a narrow range of age of onset (early adolescence), stereotypic presentation of symptoms and course, and tends to be specific to the female gender. Although often considered to be caused by psychosocial factors, recent studies have shown that genetic heritability accounts for approximately 50–80% of the risk of developing an eating disorder (ED) [2] and contributes to neurobiological factors underlying EDs [3]. A lack of understanding of the pathophysiology of these illnesses has hindered the development of effective treatments. How are individuals with AN able to consume a few hundred calories per day and maintain an extremely low weight for many years, when most people struggle to lose a few pounds? It has been controversial as to whether individuals with AN have a primary disturbance of appetite regulation or whether pathological feeding behavior is secondary to other phenomena, such as an obsessional preoccupation with body image.
Individuals with AN tend to have other puzzling symptoms and behaviors that are poorly understood, such as severe body image distortions, a lack of insight about being ill, and depriving themselves of food despite starvation (Box 1). Their disorder is egosyntonic and they often refuse or resist treatment. How are these unique behaviors encoded in neural processes? Recent studies of obesity suggest that cortico–limbic neural processes, which encode the rewarding, emotional, and cognitive aspects of food ingestion, can drive overconsumption of food, even in the presence of satiety and replete energy stores [4–6]. It has been suggested that obesity and addictions share overlapping brain circuits and monoamine systems that modulate reward sensitivity, incentive motivation, conditioning (memory/learning), impulse control (behavioral inhibition), stress reactivity, and interoceptive awareness [7]. These studies have created a body of knowledge that could be used to jump-start advances towards an understanding of the neurobiology of AN. Interestingly, AN has contrasting symptoms, such as underconsumption of food (despite being emaciated) and decreased rates of alcohol and drug abuse [8,9], which suggest differences in these neural processes compared to obesity and addiction. Although such understanding is in its infancy, we argue in this review that it is important to know how symptoms and behaviors in AN are encoded in the brain, because this is necessary to improve treatment. Because of space limitations, this review discusses selected behavioral traits in AN in relation to imaging results: first, evidence that harm avoidance (HA) is related to dopamine (DA) and serotonin (5-HT) function in AN, and second, the use of functional magnetic resonance imaging (fMRI) studies to reveal insights into the neural circuitry of gustatory sensory response, interoception, reward, and executive control.
Box 1. Example of a typical AN case
E.P. was a woman who developed AN at 13 years of age. Her parents described her as a compliant but shy child, sensitive to change and criticism. She was anxious and worried about something happening to her parents. She was a perfectionistic child who got all As and was obsessively organized in terms of her clothes and desk. Around the age of 13, after menarche (she only had one menstrual period) she began to restrict her food intake and complained about feeling fat. At this point she was 5 ft. 2 in. and weighed approximately 95 lbs. She became a vegetarian, saying that she was disgusted by eating meat, and she started to overexercise, running several miles a day. She had increased concerns about making mistakes and increased worries over perceived consequences.
Over time, she developed more severe restricting behaviors, often refusing to eat with the family, and eating small low-calorie meals with unusual food combinations (cabbage and ketchup) in a ritualized manner. Although her parents noticed that she was losing weight, they were not terribly concerned about it because she continued to do well in school, and in fact seemed even more driven towards accomplishment. When they took her to her pediatrician for a routine examination, they found she had lost more than 20 lbs and her blood pressure, pulse, and temperature were abnormally low. The pediatrician made a diagnosis of AN. E.P. was unconcerned and denied being emaciated, holding out her arm to the pediatrician and saying, ‘Can’t you see how fat I am?’ She was hospitalized because of her medical instability. After many months of inpatient treatment she gained weight, but only with much struggle, as she often stated that gaining weight made her feel fat and nothing was more important to her than being thin. After discharge, she relapsed and was rehospitalized, starting a cycle of inpatient weight gain and outpatient weight loss over the next 10 years, until her family, exhausted by the struggle, gave up forcing her into treatment. Subsequently, she moved out, refused to have anything to do with her family because of their concerns about her weight, and died of malnutrition at the age of 25.
Temperament and personality in AN
Although many individuals diet and seek to lose weight in our culture, relatively few develop AN. In fact, the prevalence is less than 1% of women [1]. Individuals with AN tend to have certain temperament and personality traits, which often first occur in childhood before the onset of an ED and may create a vulnerability to develop an ED. In addition to predating the disease, these traits often persist after recovery [10–14]. The traits include anxiety, negative emotionality, perfectionism, inflexibility, HA, and obsessive behaviors (particularly with order, exactness, and symmetry). This personality and behavioral profile may constitute an intermediate phenotype between genes and vulnerability to AN. A brief overview of some of these behaviors is given below.
Anxiety
AN is associated with high anxiety that is premorbid to the illness [11] and persists after weight restoration. This suggests an underlying anxious trait that is independent of nutritional status [13]. Comorbid anxiety disorders occur significantly more frequently in AN individuals than in control subjects, with lifetime prevalence rates of up to 50% [15]. The presence of childhood anxiety disorders predicts more severe ED symptoms, such as lower body mass index (BMI) and more psychopathology [15,16] and elevated anxiety is associated with poor outcome [17].
Inhibitory self-control and reward
Individuals with AN have long been noted to be anhedonic and ascetic, able to sustain the self-denial not only of food but also of most comforts and pleasures in life [18]. Thus, an altered balance between reward and inhibition appears to be a hallmark of AN. For example, subjects with AN have an enhanced ability to delay reward (i.e., show less reduction in the value of a monetary reward over time) compared to healthy volunteers [19]. AN subjects also have high punishment sensitivity and low reward reactivity during both the ill and recovered states [20].
Set shifting
Adult ill and recovered AN subjects, as well as their unaffected sisters, show impaired set-shifting (i.e., rigid response to changing rules and elevated perseverative responses and switching errors) [21–23], suggesting that cognitive inflexibility is a trait marker of AN. However, mixed findings for impaired set-shifting in adolescent AN call into question whether set-shifting difficulties really are a predisposing trait, a result of chronic AN, or related to a particular stage of brain development [24–26].
Harm avoidance
A considerable body of literature shows that HA, a multi-faceted temperament trait that contains elements of anxiety, inhibition, and inflexibility [27], is elevated in individuals who are ill with AN and persists after recovery [12–14,28,29]. AN adults are also less tolerant of uncertainty, and this is correlated with both HA and depression [30].
Perfectionism
Individuals with AN tend to be perfectionistic, with an overemphasis on self-imposed standards [31]. Perfectionism is a risk factor for AN; high levels of perfectionism precede the onset of AN [32] and have been associated with poor recovery and shorter duration of remission [33,34]. Moreover, levels of perfectionism do not change with a reduction in the ED and comorbid psychiatric symptoms during recovery [34]. Perfectionism may exert effects on appetite regulation because it has been shown to mediate the relationship between perceived criticism and restrictive dieting [35].
Interoception and alexithymia
Altered interoceptive awareness might be a precipitating and reinforcing factor in AN [36–38]. The role of the anterior insula in integrating interoceptive information (e.g., internal physical sensations including taste, pain, and hunger) and altered insula activity found in individuals with AN (discussed below) supports the idea that they might suffer from a fundamentally and physiologically altered sense of self [39]. Indeed, many of the symptoms of AN, such as distorted body image, lack of recognition of the symptoms of malnutrition (e.g., a failure to appropriately respond to hunger) and diminished motivation to change [40], could be related to disturbed interoceptive awareness. Such disturbances could also be tied to the preponderance of alexithymia (difficulty in identifying emotions) in AN [41,42]. Alexithymia and disrupted interoceptive processing persist even after recovery and are thought to contribute to deficits in emotional processing in AN [43].
Appetite regulation in AN
It is possible that many of the aforementioned factors contribute to pathological feeding behavior in AN. For example, there is an anxiety-reducing effect of dietary restraint and reduced daily caloric intake associated with AN [44,45], whereas food consumption stimulates dysphoric mood [46]. Moreover, enhanced inhibition, self-control, and/or an ability to delay reward may help to maintain persistent food restriction. Finally, disturbed interoceptive awareness of satiety or hunger, or even a primary alteration of primary gustatory processes, could play a role in assessing body states and responding to hunger cues.
DA and 5-HT: relationship to HA
Considerable data show that AN individuals have disturbances of DA and 5-HT systems [3]. It has been proposed that 5-HT might play a role in altered satiety, impulse control, and mood, and that DA may be implicated in aberrant rewarding effects of food, motivation, or executive functions (inhibitory control, salience attribution, and decision-making). Because of space limitations, this review focuses on measures that assess 5-HT and DA levels in the brain, such as cerebrospinal fluid (CSF) measurement of metabolites, positron emission tomography (PET), and single photon emission computed tomography (SPECT) brain imaging studies (Tables 1 and 2).
Receptor/transporter | Imaging | III | Recovered | Regions | Direction of changeb | Refs |
---|---|---|---|---|---|---|
5-HT2A | PET | – | AN | Mesial temporal (amygdala and hippocampus), pre- and subgenual cingulate, occipital/parietal cortical regions | – | [103] |
– | AN-BN | Left subgenual cingulate, left parietal cortex, right occipital cortical regions | – | [104] | ||
AN, AN-BN | – | – | = | [105] | ||
SPECT | AN | – | Left frontal, bilateral parietal, occipital cortical regions | – | [106] | |
5-HT1A | PET | n/a | AN | _ | = | [107] |
n/a | AN-BN | Cingulate, lateral and mesial temporal, lateral and medial orbital frontal, parietal, and prefrontal cortical regions; dorsal raphe | + | [107] | ||
AN, AN-BN | – | Cingulate, lateral and mesial temporal, lateral and medial orbital frontal, parietal, and prefrontal cortical regions; dorsal raphe | + | [105] | ||
– | AN | Right superior temporal, inferior frontal gyrus; parietal operculum, temporoparietal junction; left amygdala, parahippocampal gyrus, left temporal pole | + | [108] | ||
AN | n/a | Right superior temporal, inferior frontal gyrus; parietal operculum, temporoparietal junction; left amygdala, parahippocampal gyrus, left temporal pole | + | [108] | ||
5-HTT | PET | – | AN | – | = | [57] |
– | AN-BN | – | = | [57] |
Table 1. Summary of CNS 5-HT Receptor and 5-HTT PET and SPECT Studies in AN in the Ill and Recovered States Compared to Healthy Control Subjectsa
Receptor/transporter | III | Recovered | HA correlation | Regions | Refs |
---|---|---|---|---|---|
5-HT2A | – | AN | No | – | [103] |
– | AN-BN | Positive | Subgenual cingulate, mesial temporal | [104] | |
AN, AN-BN | Positive | Supragenual cingulate, frontal, parietal | [105] | ||
5-HT1A | – | AN | Positive | Subgenual cingulate, mesial temporal | [107] |
– | AN-BN | No | – | [107] | |
DA D2/D3 | – | AN, AN-BN | Positive | Dorsal caudate, dorsal putamen | [18] |
– | AN, AN-BN, BN | Positive | Dorsal caudate, dorsal putamen | [47] |
Table 2. Correlations of 5-HT and DA D2/D3 Receptor Binding and HA in Recovered and Ill AN, as Measured With PET Imaging
Alterations in the dopaminergic system
Using the radioligand [11C]raclopride, several PET studies have found that recovered AN subjects have increased binding of DA D2/D3 receptors in the anterior ventral striatum relative to control subjects (Figure 1a) [18,47]. Because PET measurements of [11C]raclopride binding are sensitive to endogenous DA concentrations [48], this difference could be due to either a reduction in the intrasynaptic DA concentration or elevation of the density and/or affinity of the D2/D3 receptors in this region. The former hypothesis is supported by a finding that recovered AN individuals have lower levels of the DA metabolite homo-vanillic acid (HVA) in CSF compared to control women [49].
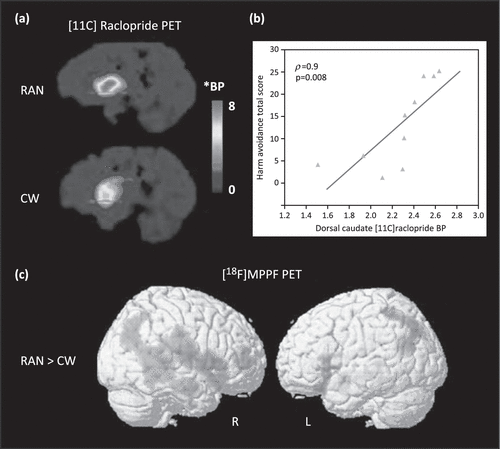
Figure 1. Positron Emission Tomography (PET) Studies Show Altered Dopamine (DA) and Serotonin (5HT) Function in Anorexia Nervosa (AN).
(a) Comparison of DA D2/D3 Receptor Binding in One Recovered AN (RAN) and One Age-Matched Control Woman (CW). The Red line Indicates the Level of the Right Anteroventral Striatum; BP, Binding Potential. *BP = [(Region of Interest/Cerebellum/−1]. Reproduced, With Permission, from [18]. (b) Correlation (Spearman Rho Coefficient) Between the Harm Avoidance Total Score and Dorsal Caudate [11C]Raclopride BP in RAN Subjects. The p Value is Bonferroni-Corrected. Reproduced, With Permission, from [18]. (c) Statistical Parametric Mapping Analysis of 4-(2-Methoxyphenyl)-1-[2-(N-2-Pyridinyl)-p-Fluorobenzamido]-Ethylpiperazine ([18F]MPPF) Binding for Comparison of Clinically Recovered AN (RAN) and Control Subjects. Increased 5-HT1A Binding (Represented by Red) is Seen in Recovered and Lean (not Shown) AN in Widespread Regions Including the Right Temporofrontal Cortex, Amygdala–Parahippocampal Complex, and Temporoparietal Junction. Reproduced, With Permission, from [108].
Alterations in the serotonergic system
PET and SPECT studies have assessed 5-HT1A and 5-HT2A receptors and the 5-HT transporter (5-HTT) in AN [50]. Most, but not all, studies show that ill and recovered individuals with AN tend to have increased 5-HT1A binding (Figure 1c) and reduced 5-HT2A binding (Table 1). 5-HT2A and 5-HT1A postsynaptic receptors are highly colocalized (∼80%) in the rodent frontal cortex [51] and other cortical regions [52]. Through interneurons, they mediate direct hyperpolarizing and depolarizing actions of 5-HT on pre-frontal neurons that project to cortical and subcortical areas [53,54]. This leads to the interesting speculation that exaggerated 5-HT1A versus diminished 5-HT2A could result in hyperpolarizing effects on prefrontal neurons in AN. Interactions between 5-HT1A and 5-HT2A receptors in the medial prefrontal cortex (mPFC) and related regions have been found to modulate anxiety, attentional functioning [55], impulsivity and compulsive perseveration [54], and exploration of novel environments [56]. Differences in 5-HTT function could contribute to differences in impulse control, and thus explain why individuals develop AN (of the restricting type) rather than AN-bulimic type (AN-BN). Whereas 5-HTT levels in AN and AN-BN individuals are comparable to controls (Table 1), 5-HTT differences have been observed when AN and AN-BN subgroups are compared [57]. These findings may be consistent with a recent study showing that the S allele of the 5HTTLPR (serotonin-transporter-linked polymorphic region in SLC6A4) genotype increases the risk susceptibility for diagnostic crossover over time in ill subjects [58].
Relationship between 5-HT, DA, and HA
Many of the PET studies carried out show striking and consistent correlations between the binding potential of both 5-HT1A or 5-HT2A, DA D2/D3 (Figure 1b), and HA in cingulate, mesial temporal, and parietal regions (Table 2). Although the mechanisms by which 5-HT1A and 5-HT2A receptor function are related to HA remain uncertain, it is still possible to speculate how restricted eating may be one means of reducing anxiety. Recovered individuals showed increased CSF levels of 5-hydroxyindoleacetic acid, the major brain metabolite of 5-HT [59], whereas those who were ill with AN had reduced concentrations [60]. One study showed that depletion of tryptophan (the precursor of 5-HT), which results in a reduction in 5-HT activity, decreased anxiety in AN [61]. The 5-HT system consists of 14 or more receptors and integration of many other neurochemicals. Whether HA is solely related to 5-HT1A and 5-HT2A receptors or, in all likelihood, involves more complex mechanisms remains beyond our ability to determine using current technology.
HA is also positively associated with dorsal caudate DA D2/D3 binding potential in recovered AN/AN-BN (Table 2) [18,47]. Consistent with this finding, an fMRI study showed that baseline trait anxiety was positively correlated with dorsal caudate blood oxygen-level-dependent (BOLD) responses to positive and negative feedback [62]. These findings are consistent with studies in rats showing that D2 receptors within the dorsomedial striatum are associated with response inhibition [63], and that rats characterized as risk-averse have greater D2 mRNA expression within the dorsal striatum [64]. This suggests that DA signaling within executive corticostriatal circuitry may reflect a heightened response to adverse consequences and/or increased inhibitory control [65]. These data support the provocative possibility that aberrant function of the dorsal caudate creates a vulnerability towards developing anxious, inhibited, shy, inflexible, and risk-averse behaviors, traits that are often observed in childhood before the onset of AN.
DA, anxiety, and restricted eating
To further explore DA function and behaviors, a recent study used PET [11C]raclopride binding with amphetamine to assess endogenous DA release [66]. This study confirmed that DA release in the precommissural dorsal caudate was associated with increased anxiety in recovered subjects, whereas control subjects showed the well-known euphoria associated with anterior ventral striatum DA release. Ingestion of palatable food is associated with striatal endogenous DA release [67,68]. If AN individuals experience endogenous DA release as anxiogenic rather than hedonic, this may explain their pursuit of starvation, because food refusal may be an effective means of diminishing the anxious feelings associated with the disorder.
Competition between DA and 5-HT
It has been theorized that 5-HT is the crucial substrate of an aversive motivational system that might oppose a DA-related appetitive system [69,70]. That is, 5-HT has a critical role in the adaptation of animals to aversive events [71,72] and may mediate a negative prediction error signal for future threat and punishment [69,70]. Although DA has been associated with the expression of an appetitive reward system [73], it is plausible that it works in mutual opponency with a system that signals the prediction of punishment instead of reward [69,72]. From another perspective, studies suggest that 5-HT has a role in action choice by controlling the timescale of delayed rewards through differential effects on ventral and dorsal striatal circuits [74,75]. We hypothesize that AN individuals have a temperament skewed towards aversive or inhibitory response rather than reward and motivation (Figure 2). It is important to note that 5-HT and DA neural systems are complex. For example, the 5-HT system has 14 or more receptors and many other components that modulate metabolism, firing rate, and neuronal cascades, among other functions. However, there is evidence supporting the possibility that interactions between 5-HT and DA contribute to temperament in AN. For instance, a PET study of a mixed group of subjects with recovered ED showed a significant positive correlation between 5-HTT and DA D2/D3 binding for the dorsal caudate, antero–ventral striatum, medial caudate, ventral putamen, and dorsal putamen [47]. Linear regression analysis showed that the interaction between 5-HTT and DA D2/D3 binding potential in the dorsal putamen significantly predicted HA, with a trend towards significance in the dorsal caudate.
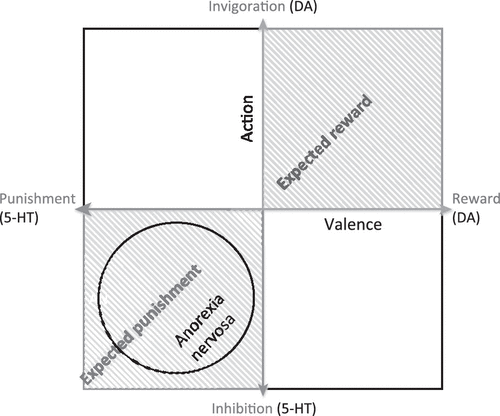
Figure 2. Hypothetical Model Illustrating the Competition/Cooperation Between Dopamine (DA) and Serotonin (5-HT) and the Implications for Anorexia Nervosa (AN).
This Model is Based on Previous Models [109–112] and Shows the Hypothetical Interaction Between 5-HT Functional Activity (Aversive or Inhibitory) and DA Functional Activity (Reward or Motivation) [69,70]. Measurements of Cerebrospinal Fluid Metabolites Suggest that Recovered AN Subjects have Increased Brain 5-HT [59] and Decreased Brain DA [49]. This Suggests that Individuals With AN may have a Temperament that places them in the Lower-Left Quadrant of this Model, Supporting the Hypothesis of a Skew Towards Aversive or Inhibitory Responses Rather than Reward and Motivation.
A substantial limitation of neurotransmitter studies in humans is that only a few neuromodulatory components can be measured, and there are currently no means to measure or model the functional interactions of the many biochemical mechanisms that make up these complex systems. Although an understanding of the molecular mechanisms remains far beyond the abilities of technologies available for studies in humans, these are still potentially valuable insights. As yet, there are no medications or other treatments that have been proven to reverse core symptoms in AN. These findings may help to identify drugs that act on 5-HT and/or DA neural processes that may be best suited to reduce HA behaviors in AN. For example, it has been debated whether olanzapine, a drug with 5-HT and DA effects, is useful for weight gain in AN [76,77], although such a drug may be effective in diminishing anxiety in AN patients [78].
fMRI studies of appetite: relationship to food taste
fMRI imaging studies of appetitive behaviors in ED have used designs that either administer a taste of some food or present images of food to participants. The neurocircuitry of taste processing in healthy individuals is well understood [5,7,79]. The anterior insula is the primary gustatory taste cortex and responds to tastes of food [3]. The anterior insula, as well as the anterior cingulate cortex and orbital frontal cortex, code the sensory–hedonic response to taste and innervate a broad region of the rostral ventral–central striatum, where behavioral repertoires are then computed. This network may play a crucial role in linking sensory–hedonic experiences to the motivational components of reward and emotionality, providing conscious awareness of these urges. This review focuses on studies that administer tastants and the interrogation of the circuitry using this method. Although equally valuable, space limitations preclude a discussion of response to food images [80,81].
Although there have been relatively few tastant studies in AN, the literature to date is relatively consistent between the ill and recovered states, supporting the possibility that altered reward and interoceptive processing are trait characteristics of the disorder. In one of the first studies that tested the reward processes behind interoceptive stimuli, tastes of sucrose and water were administered to recovered AN subjects (Figure 3a) [82]. Compared to control women, recovered AN subjects showed lower neural activation of the ventral and dorsal striatum, as well as the insula (including the primary cortical taste region), to both sucrose and water. Moreover, insular neural activity correlated with pleasantness ratings for sucrose in control but not AN subjects (Figure 3b). In a more recent study, hunger and satiety states were compared in ill AN and control subjects when drinking chocolate milk [83]. In the fasting state, the insula was significantly activated in response to drinking chocolate milk in control subjects but not ill AN individuals. These findings suggest minimal insula response in AN to the tastes of food when hungry. By contrast, imaging studies in healthy individuals have consistently shown that food deprivation, when compared to states of satiety, activates the insula and the orbital frontal cortex [84]. Considered together, these findings raise the intriguing possibility that AN subjects have an altered setpoint and/or altered sensitivity for sensory–interoceptive–reward processes when consuming palatable foods [79]. Thus, the setpoint may mimic a continuous state of satiety in individuals with AN that limits interoceptive and reward processing.
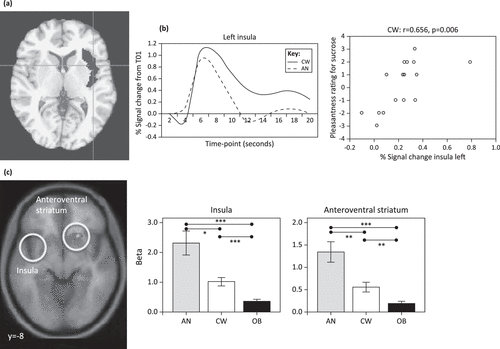
Figure 3. Altered Neurocircuitry of Taste Consumption and Anticipation in Anorexia Nervosa (AN) as Revealed by Functional Magnetic Resonance Imaging (fMRI).
(a) A Decrease in Taste-Related (Sucrose and Water) Blood Oxygen-Level-Dependent (BOLD) Response was Found for Recovered AN Compared to Control Women (CW) in the Left Insula Region of Interest (ROI; Highlighted in Gray in Axial View) and Left Ventral Putamen (not Shown). The Corresponding Time Course of the BOLD Signal is Shown as a Mean for 16 AN and 16 CW Subjects in the Left Insula ROI. This Suggests that in AN the Insula may not Accurately Encode Gustatory Signals, Perhaps as Part of a More Widespread Defect in Interoceptive Awareness or Perhaps Encompassing Altered Ability to Gauge Satiety or Hunger Setpoints. Insula Responses may also be Modulated by Reward Determination (e.g., in Anterior Ventral Striatum Circuits, Specifically the Nucleus Accumbens), which may Fail to Properly Recognize, Scale, or Modulate Reward Response in AN. Reproduced, With Permission, from [82]. (b) In Support of Altered Interoceptive/Reward Processing, BOLD Response is Correlated With Pleasantness Rating for Sucrose for CW in the Left and Right (not Shown) Insula but not for Recovered AN (not Shown). Reproduced, With Permission, from [82]. (c) In Response to a Taste Reward Conditioning Task that has been Associated With Activation of Dopamine Reward Circuits, Computational Model-Derived Data Revealed Ill Underweight AN Subjects had Greater Brain Response During Anticipation of Taste In the Anteroventral Striatum, Insula, and Prefrontal Cortex Compared to Control Women and Obese (OB) Women. These Results Suggest that Brain Reward Circuits are More Responsive to Food Stimuli in AN, But Less Responsive in Obese Women. The Mechanism for this Association is Uncertain, but these Brain Reward Response Patterns Could be Biomarkers for the Corresponding Weight State. Reproduced, With Permission, from [86].
Previous studies of obese subjects have highlighted the importance of anticipation in the neural response to food stimuli [85]. Animal studies show that following stimulus conditioning, DA neuron firing shifts from the consumption of food to the anticipated consumption of food or to cues associated with food consumption [85,86]. A number of studies used tastants in ED in which the design might elicit anticipatory responses. For instance, a recent study performed an associative learning task between conditioned visual stimuli and unconditioned sucrose taste stimuli in ill AN, control, and obese participants (Figure 3c) [86]. Reward learning signals in the anterior ventral striatum, insula, and the orbitofrontal cortex (OFC) were greater in the AN subjects and less in the obese participants compared to control subjects. These findings led to the conclusion that brain reward circuits are more responsive in AN and less responsive in obese participants. Another study used a complex design in which recovered AN and control participants viewed and tasted pleasant and unpleasant stimuli either alone or paired together [87]. Recovered AN subjects had increased ventral striatal activity in response to sights and flavors of a pleasant stimulus (chocolate) and increased insula and posterior dorsal caudate response to flavors and sights of aversive foods compared to control subjects. Taken together, these studies suggest that AN subjects may be highly responsive to stimulus cues, perhaps as a mechanism to predict and control the anxiety produced by stimuli that may be associated with subjective unpleasantness. This type of anticipatory sensitivity connected with stimulus avoidance has been observed in highly anxious individuals [88].
The imbalance between stimulus consumption and anticipation in AN may suggest dysfunctional stimulus integration that could relate to the clinically observed disconnect between reported and actual interoceptive states. Key in this process is the insula. This brain region is thought to code interoceptive prediction error, signaling mismatch between actual and anticipated bodily arousal, which in turn elicits subjective anxiety and avoidance behavior [89]. A study of response to pain confirms a mismatch between anticipation and objective responses in recovered AN individuals [42]. In this study, recovered AN compared to control participants showed greater activation within the right anterior insula, dorsolateral pre-frontal cortex (DLPFC), and cingulate cortex during pain anticipation, and greater activation within the DLPFC and decreased activation within the posterior insula during painful stimulation. Greater anticipatory anterior insula activation correlated positively with alexithymia (difficulty in identifying emotions) in recovered AN subjects. Alexithymia assessment provided additional evidence of an altered ability to accurately perceive or predict bodily signals, which persists even after recovery. Given that food is an inherently emotional stimulus in AN, a mismatch between limbic hyperarousal and executive control may be tied to interoceptive and/or emotional processing deficits in this population.
fMRI studies of reward and executive control
A series of fMRI studies using response to reward or executive control tasks has explored the role of ventral and dorsal corticostriatal systems in AN in comparison to control subjects to better understand the modulation of reward, emotionality, and behavioral inhibition. Control subjects show patterns of limbic striatal response that significantly distinguish positive and negative feedback [90]. By contrast, another study reported that recovered AN subjects had minimal differences to positive and negative feedback in limbic striatal regions and an exaggerated response in the dorsal caudate and associated executive regions relative to control women [62]. Interestingly, and in support of a relationship between anxiety and dorsal caudate DA findings (as described above), dorsal caudate activity was positively associated with baseline trait anxiety for both positive and negative feedback in AN [62]. In another study that used a set-shifting fMRI paradigm, ill AN subjects showed predominant activation of frontoparietal networks, indicative of excessive effortful and supervisory cognitive control during task performance, but hypoactivation in the ventral anterior cingulated–striato–thalamic loop involved in motivation-related behavior [91].
Other studies using different tasks have found evidence of abnormal function in executive circuitry in AN. For example, using a Go/No Go task, AN-BN adolescents showed greater activation than control subjects in the anterior cingulate cortex, and greater activation compared to both control subjects and AN adolescents in the right DLPFC [92]. Furthermore, AN participants showed a positive correlation between percentage correct on No Go trials and activation in inferior parietal cortex regions. Another study, using a stop signal task, found that recovered AN subjects had altered task-related activation in the mPFC, a critical node of the inhibitory control network [93].
These data support the hypothesis that AN individuals have an imbalance within and/or between ventral limbic and dorsal executive circuits [3]. Specifically, a ventral limbic neural circuit (which includes the amygdala, anterior insula, anterior ventral striatum, ventral regions of the anterior cingulate cortex, and the OFC) is involved in identifying rewarding and emotionally significant stimuli required to generate affective responses to these stimuli [94,95]. The anterior ventral striatum processes motivational aspects of stimuli by modulating the influence of limbic inputs on striatal activity [96,97]. Decreased anterior ventral striatum responses to cues may reflect a failure to appropriately bind, scale, or discriminate responses to salient stimuli. This supports the possibility that recovered AN subjects may have an impaired ability to identify the emotional significance of stimuli.
A dorsal executive-function neural circuit (which includes dorsal regions of the caudate, DLPFC, parietal cortex, and other regions) is thought to modulate selective attention, planning, and effortful regulation of affective states. The ventral limbic and dorsal executive neural circuits are critically involved in inhibitory decision-making processes, especially involving reward-related behaviors. Together they process the reward value and/or affective valence of environmental stimuli, assess the future consequences of one’s own actions (response selection), and inhibit inappropriate behaviors (response inhibition). It has been proposed that dysfunction within these regions is a key neural mechanism underlying altered behavioral regulation, reward regulation, and cognition found in drug addiction [98,99]. In summary, individuals with AN may have an imbalance in information processing, with impaired ability to identify the emotional significance of a stimulus, but increased traffic in neurocircuits concerned with planning and consequences leading to heightened anxiety. This over-reliance on executive brain circuits involved in linking action to outcome may constitute an attempt at strategic (as opposed to hedonic) means of responding to reward stimuli [100]. These studies provide further evidence that exaggerated dorsal executive function is associated with enhanced inhibition and may reflect a possible means of modulating anxiety in AN.
Concluding remarks
Considerable evidence shows that individuals with AN have anxious, inhibited, and inflexible premorbid characteristics. It is possible that these premorbid traits are related to altered monoamine neuronal modulation, or dorsal caudate function, that predates the onset of AN. Several factors might exacerbate these vulnerabilities to cause the onset of AN in adolescence. First, puberty-related female gonadal steroids might exacerbate 5-HT and DA system dysregulation [101]. Brain changes associated with puberty might further challenge these processes. For example, increased activity of orbital and DLPFC regions during and after puberty might contribute to excessive worry, perfectionism, and strategizing in AN patients [102]. Finally, stress and/or cultural and societal pressures might contribute by increasing anxious and obsessional temperament. However, relatively little work has been done to investigate the psychobiology of this disorder compared to other severe psychiatric disorders, leaving many questions still unanswered (Box 2).
Box 2. Outstanding questions
• | Why is it important to understand the neurobiology of disturbed behavior in AN? Several potential hypotheses might explain why AN individuals are perfectionistic. They may have poor prediction certainty, as evidenced by alexithymia. Alternatively, they may have exaggerated responses to negative feedback and a diminished response to reward, so they perceive their world as constantly having errors. New treatments could be developed to teach skills to overcome these traits. However, these two hypotheses might require very different psychological interventions. Thus, an understanding of how perfectionistic behavior is manifested and encoded in neural circuits may be necessary to develop specific and effective treatments. | ||||
• | State and trait in AN. Cause and effect between pathological eating and the impact of malnutrition on neural processes in AN remain a major methodological question. Malnutrition in AN is associated with changes in brain structure (e.g., reduction in gray matter, altered white matter integrity) and profound metabolic, electrolyte, and endocrine disturbances [113–117]. Studies in animals suggest that diet and weight can influence DA [118,119] and 5-HT metabolism [61]. Strategies to avoid the confounding effects of abnormal nutritional status include: (i) characterizing behavioral traits that occur in childhood, prior to the onset of an ED, and (ii) studying recovered anorexics, although it remains conjectural whether abnormal findings reflect traits or scars. | ||||
• | AN subtypes. Restricting-type anorexics (AN) lose weight purely by restricted dieting. Overtime, up to 50% of these individuals will also develop purge and/or binge behaviors (AN-BN) whereas the rest remain pure restrictors [120]. Many studies do not differentiate between these subtypes. Currently, the neurobiological factors that differ between the subtypes or are associated with transitioning between the subtypes are not well understood. One possibility is that the subtypes have differential effects on impulse control modulation [121]. | ||||
• | What is the neural circuitry underlying AN? Understanding how puzzling AN behavior is encoded in neural circuitry is a major methodological challenge for the ED field. Several fMRI strategies can be used to investigate brain and behavior. One is to provoke AN symptoms (e.g., body image distortions) and determine what regions are abnormal. Alternatively, a focus on tasks designed to interrogate reward processing (e.g., response to positive and negative feedback) can be used to determine differences in a priori identified neural circuitry. Both methods are valuable. However, symptoms associated with AN (e.g., body image distortion) could be secondary, and serve to make sense of other powerful aberrant drives. We think it is critical to understand how premorbid temperament traits are encoded in brain circuits, because these may be key traits essential for developing AN. | ||||
• | What about body image distortion? This may be the most puzzling of all AN symptoms, in part because AN individuals feel fat but tend to have normal perceptions of other people’s bodies. A recent review concluded that the perceptive component was related to alterations of the precuneus and the inferior parietal lobe, and the affective component was related to alterations of the prefrontal cortex, the insula, and the amygdala [122]. Although the mechanism remains obscure, a recent study points to impaired integration of visual and proprioceptive information [123]. |
AN individuals tend to find that restricting food intake provides a temporary respite from dysphoric mood. People with AN may enter a vicious cycle – which could account for the chronicity of this disorder – because eating exaggerates, and food refusal reduces, an anxious mood. Imaging studies of neural processes are in their infancy and molecular mechanisms remain unexplored. However, studies that have investigated monoamine function support the hypothesis that AN temperament and personality might be related to exaggerated performance of serotonin systems that mediate a negative prediction error signal for future threat and punishment and diminished effects of DA appetitive reward systems. From another perspective, fMRI studies suggest there are disturbances of higher-order circuits related to interoception, reward, emotionality, and inhibition that regulate approach and avoidance of food. However, cause and consequence remain unclear. For example, it is possible that hyperactive executive circuits directly motivate actions when the ability of the ventral striatal pathways to direct more automatic or intuitive motivated responses is impaired. Another possibility is that in AN patients, (otherwise adequate) limbic–striatal information-processing in the ventral circuit is too strongly inhibited by converging inputs from executive domains. Moreover, diminished reward combined with increased executive control could contribute to alexithymia through the suppression or misinterpretation of perturbed body signals from the insula.
It has been difficult to modify core traits of ED in treatment settings. New insights into the neurobiology of personality and temperament in AN hold promise in this regard. First, they may inform the development of medications targeting the biology of AN that contributes to certain symptoms, such as anxiety. Although this may not be a cure for AN, it may substantially improve the ability to eat and maintain weight. Second, they may guide the development of more effective psychotherapies to elicit behavioral changes that are educated by robust and neurally derived principles of the internal mechanisms of AN.
1
2 . (2006) Prevalence, heritability and prospective risk factors for anorexia nervosa. Arch. Gen. Psychiatry 63, 305–312Crossref, Google Scholar
3 . (2009) New insight into symptoms and neurocircuit function of anorexia nervosa. Nat. Rev. Neurosci. 10, 573–584Crossref, Google Scholar
4 . (2006) Homeostatic and non-homeostatic pathways involved in the control of food intake and energy balance. Obesity 14 (Suppl. 5), 197S–200SCrossref, Google Scholar
5 . (2006) Brain mechanisms underlying flavour and appetite. Philos. Trans. R. Soc. Lond. B: Biol. Sci. 361, 1123–1136Crossref, Google Scholar
6 . (2006) Central gustatory processing in humans. Adv. Otorhinolaryngol. 63, 191–220Google Scholar
7 . (2012) Food and drug reward: overlapping circuits in human obesity and addiction. Curr. Top. Behav. Neurosci. 11, 1–24Crossref, Google Scholar
8 . (2009) Meta-analysis on drugs in people with eating disorders. Eur. Eat. Disord. Rev. 17, 243–259Crossref, Google Scholar
9 . (2007) Co-occurrence of eating disorders and alcohol use disorders in women: a meta analysis. Arch. Womens Ment. Health 10, 133–140Crossref, Google Scholar
10 . (2003) Childhood obsessive–compulsive personality traits in adult women with eating disorders: defining a broader eating disorder phenotype. Am. J. Psychiatry 160, 242–247Crossref, Google Scholar
11 . (2004) Comorbidity of anxiety disorders with anorexia and bulimia nervosa. Am. J. Psychiatry 161, 2215–2221Crossref, Google Scholar
12 . (2005) Personality and eating disorders: a decade in review. Clin. Psychol. Rev. 25, 895–916Crossref, Google Scholar
13 . (2006) Personality traits after recovery from eating disorders: do subtypes differ? Int. J. Eat. Disord. 39, 276–284Crossref, Google Scholar
14 . (2011) Personality and temperament. In Behavioral Neurobiology of Eating Disorders (Current Topics in Behavioral Neurosciences, Vol. 6) (Kaye W.H, Adan R, eds), pp. 3–16, SpringerGoogle Scholar
15 . (2008) Influence of overanxious disorder of childhood on the expression of anorexia nervosa. Int. J. Eat. Disord. 41, 326–332Crossref, Google Scholar
16 . (2010) Childhood anxiety associated with low BMI in women with anorexia nervosa. Behav. Res. Ther. 48, 60–67Crossref, Google Scholar
17 . (2011) Genetic association of recovery from eating disorders: the role of GABA receptor SNPs. Neuropsychopharmacology 36, 2222–2232Crossref, Google Scholar
18 . (2005) Increased dopamine D2/D3 receptor binding after recovery from anorexia nervosa measured by positron emission tomography and [11C]raclopride. Biol. Psychiatry 58, 908–912Crossref, Google Scholar
19 . (2012) Fear of food as a treatment target: exposure and response prevention for anorexia nervosa in an open series. Int. J. Eat. Disord. 45, 615–621Crossref, Google Scholar
20 . (2010) Sensitivity to reward and punishment in eating disorders. Psychiatry Res. 177, 1–11Crossref, Google Scholar
21 . (2011) Cognitive-behavioral flexibility in anorexia nervosa. In Behavioral Neurobiology of Eating Disorders (Current Topics in Behavioral Neurosciences, Vol. 6) (Kaye W.H, Adan R, eds), pp. 111–123, SpringerGoogle Scholar
22 . (2010) Exploring the neurocognitive signature of poor set-shifting in anorexia and bulimia nervosa. J. Psychiatr. Res. 44, 964–970Crossref, Google Scholar
23 . (2012) Altered social hedonic processing in eating disorders. Int. J. Eat. Disord. 45, 962–969Crossref, Google Scholar
24 . (2011) Restrictive anorexia nervosa and set-shifting in adolescents: a biobehavioral interface. J. Adolesc. Health 49, 99–101Crossref, Google Scholar
25 . (2012) Altered implicit category learning in anorexia nervosa. Neuropsychology 26, 191–201Crossref, Google Scholar
26 . (2012) Set-shifting among adolescents with anorexia nervosa. Int. J. Eat. Disord. 45, 909–912Crossref, Google Scholar
27 . (1994) The Temperament and Character Inventory (TCI): A Guide to its Development and Use, pp. 19–28, Center for Psychobiology of PersonalityGoogle Scholar
28 . (2004) Temperament and character in eating disorders: ten years of studies. Eat. Weight Disord. 9, 81–90Crossref, Google Scholar
29 . (2004) Personality characteristics of women before and after recovery from an eating disorder. Psychol. Med. 34, 1407–1418Crossref, Google Scholar
30 . (2012) Heightened fear of uncertainty in anorexia and bulimia nervosa. Int. J. Eat. Disord. 45, 227–232Crossref, Google Scholar
31 . (2011) Avoidance of affect in the eating disorders. Eat. Behav. 12, 90–93Crossref, Google Scholar
32 . (2012) An examination of early childhood perfectionism across anorexia nervosa subtypes. Int. J. Eat. Disord. 45, 800–807Crossref, Google Scholar
33 . (2007) Hormonal and psychological factors linked to the increased thermic effect of food in malnourished fasting anorexia nervosa. J. Clin. Endocrinol. Metab. 92, 1623–1629Crossref, Google Scholar
34 . (2008) A longitudinal study of perfectionism in adolescent onset anorexia nervosa-restricting type. Eur. Eat. Disord. Rev. 16, 386–394Crossref, Google Scholar
35 . (2011) Perfectionism as a mediator between perceived criticism and eating disorders. Eat. Weight Disord. 16, e37–e44Crossref, Google Scholar
36 . (1962) Perceptual and conceptual disturbances in anorexia nervosa. Psychosom. Med. 24, 187–194Crossref, Google Scholar
37 . (2004) Clinical, psychopathological and personality correlates of interoceptive awareness in anorexia nervosa, bulimia nervosa and obesity. Psychopathology 37, 168–174Crossref, Google Scholar
38 . (2006) Eating disorders and personality: a methodological and empirical review. Clin. Psychol. Rev. 26, 299–320Crossref, Google Scholar
39 . (2008) Reduced perception of bodily signals in anorexia nervosa. Eat. Behav. 9, 381–388Crossref, Google Scholar
40 . (2008) The fault is not in her parents but in her insula – a neurobiological hypothesis of anorexia nervosa. Eur. Eat. Disord. Rev. 16, 355–360Crossref, Google Scholar
41 . (1998) The relationship between alexithymia, depression, and axis II psychopathology in eating disorder inpatients. Int. J. Eat. Disord. 23, 277–286Crossref, Google Scholar
42 . (2013) Altered insula activation during pain anticipation in individuals recovered from anorexia nervosa: evidence of interocetive dysregulation. Int. J. Eat. Disord. 46, 23–33Crossref, Google Scholar
43 . (2010) Differential modulation of valence and arousal in high-alexithymic and low-alexithymic individuals. Neuroreport 21, 998–1002Google Scholar
44 . (2003) Neurobiology of eating disorders. In Pediatric Psychopharmacology, Principles & Practice (Martin A, , eds), pp. 224–237, Oxford University PressGoogle Scholar
45 . (2010) Pre-meal anxiety and food intake in anorexia nervosa. Appetite 55, 214–218Crossref, Google Scholar
46 . (2012) Current status of functional imaging in eating disorders. Int. J. Eat. Disord. 45, 723–736Crossref, Google Scholar
47 . (2012) Interaction between serotonin transporter and dopamine D2/D3 receptor radioligand measures is associated with harm avoidant symptoms in anorexia and bulimia nervosa. Psychiatry Res. Neuroimaging http://dx.doi.org/10.1016/j.pscychresns.2012.06.010Google Scholar
48 . (2001) Amphetamine-induced dopamine release in human ventral striatum correlates with euphoria. Biol. Psychiatry 49, 81–96Crossref, Google Scholar
49 . (1999) Altered dopamine activity after recovery from restricting-type anorexia nervosa. Neuropsychopharmacology 21, 503–506Crossref, Google Scholar
50 . (2011) Serotonin: imaging findings in eating disorders. In Behavioral Neurobiology of Eating Disorders (Current Topics in Behavioral Neurosciences, Vol. 6) (Kaye W.H, Adan R, eds), pp. 59–79, SpringerGoogle Scholar
51 . (2004) Co-expression and in vivo interaction of serotonin1A and serotonin2A receptors in pyramidal neurons of prefrontal cortex. Cereb. Cortex 14, 281–299Crossref, Google Scholar
52 . (2004) Autoradiographic distribution of serotonin transporters and receptor subtypes in human brain. Hum. Brain Mapp. 22, 246–260Crossref, Google Scholar
53 . (2004) Expression of serotoinin1A and serotonin2A receptor in pyramidal and GABAergic neurons of the rat prefrontal cortex. Cereb. Cortex 14, 1100–1109Crossref, Google Scholar
54 . (2006) Dissociable contribution of 5-HT1A and 5-HT2A receptors in the medial prefrontal cortex to different aspects of executive control such as impulsivity and compulsive perseveration in rats. Neuropsychopharmacology 31, 757–767Crossref, Google Scholar
55 . (2003) Intra-prefrontal 8-OH-DPAT and M100907 improve visuospatial attention and decrease impulsivity on the five-choice serial reaction time task in rats. Psypchopharmology 167, 304–314Crossref, Google Scholar
56 . (1998) Evidence for a functional interaction between 5-HT1A and 5-HT2A receptors in rats. Psychopharmacology 140, 69–74Crossref, Google Scholar
57 . (2007) Serotonin transporter binding after recovery from eating disorders. Psychopharmacology 195, 315–324Crossref, Google Scholar
58 . (2012) Association between serotonin transporter gene polymorphism and eating disorders outcome: a 6-year follow-up study. Am. J. Med. Genet. B: Neuropsychiatr. Genet. 159b, 491–500Crossref, Google Scholar
59 . (1991) Altered serotonin activity in anorexia nervosa after long-term weight restoration. Does elevated cerebrospinal fluid 5-hydroxyindoleacetic acid level correlate with rigid and obsessive behavior? Arch. Gen. Psychiatry 48, 556–562Crossref, Google Scholar
60 . (1984) Abnormalities in CNS monoamine metabolism in anorexia nervosa. Arch. Gen. Psychiatry 41, 350–355Crossref, Google Scholar
61 . (2003) Anxiolytic effects of acute tryptophan depletion in anorexia nervosa. Int. J. Eat. Disord. 33, 257–267Crossref, Google Scholar
62 . (2007) Altered reward processing in women recovered from anorexia nervosa. Am. J. Psychiatry 164, 1842–1849Crossref, Google Scholar
63 . (2011) Contrasting roles for dopamine D1 and D2 receptor subtypes in the dorsomedial striatum but not the nucleus accumbens core during behavioral inhibition in the stop-signal task in rats. J. Neurosci. 31, 7349–7356Crossref, Google Scholar
64 . (2011) Dopaminergic modulation of risky decision-making. J. Neurosci. 31, 17460–17470Crossref, Google Scholar
65 . (2012) Striatal dopamine D2/D3 receptors mediate response inhibition and related activity in frontostriatal neural circuitry in humans. J. Neurosci. 32, 7316–7324Crossref, Google Scholar
66 . (2012) Amphetamine induced dopamine release increases anxiety in individuals recovered from anorexia nervosa. Int. J. Eat. Disord. 45, 263–271Crossref, Google Scholar
67 . (2012) Dysregulation of brain reward systems in eating disorders: neurochemical information from animal models of binge eating, bulimia nervosa, and anorexia nervosa. Neuropharmacology 63, 87–96Crossref, Google Scholar
68 . (1999) Differential responsiveness of dopamine transmission to food-stimuli in nucleus accumbens shell/core compartments. Neuroscience 89, 637–641Crossref, Google Scholar
69 . (2002) Opponent interactions between serotonin and dopamine. Neural Netw. 15, 603–616Crossref, Google Scholar
70 . (2008) Serotoninergic regulation of emotional and behavioural control processes. Trends Cogn. Sci. 12, 31–40Crossref, Google Scholar
71 . (2010) Serotonin modulates sensitivity to reward and negative feedback in a probabilistic reversal learning task in rats. Neuropsychopharmacology 35, 1290–1301Crossref, Google Scholar
72 . (1991) 5-HT and mechanisms of defense. J. Psychopharmacol. 5, 305–315Crossref, Google Scholar
73 . (1997) Dopamine neurons and their role in reward mechanisms. Curr. Opin. Neurobiol. 7, 191–197Crossref, Google Scholar
74 . (2007) Serotonin and the evaluation of future rewards: theory, experiments, and possible neural mechanisms. Ann. N. Y. Acad. Sci. 1104, 289–300Crossref, Google Scholar
75 . (2004) Separate neural systems value immediate and delayed monetary rewards. Science 306, 503–507Crossref, Google Scholar
76 . (2012) Antipsychotic agents in the treatment of anorexia nervosa: Neuropsychopharmacologic rationale and evidence from controlled trials. Curr. Psychiatry Rep. 14, 398–405Crossref, Google Scholar
77 . (2012) The effect of atypical antipsychotic medications in individuals with anorexia nervosa: a systematic review and meta-analysis. Int. J. Eat. Disord. http://dx.doi.org/10.1002/eat.22059Google Scholar
78 . (2003) Olanzapine treatment of anorexia nervosa: a retrospective study. Int. J. Eat. Disord. 33, 234–237Crossref, Google Scholar
79 . (2009) Individual differences in the neurophysiology of reward and the obesity epidemic. Int. J. Obes. 33 (Suppl. 2), S44–S48Crossref, Google Scholar
80 . (2011) Attentional processing of food pictures in individuals with anorexia nervosa – an eye-tracking study. Biol. Psychiatry 69, 661–667Crossref, Google Scholar
81 . (2011) Differential neural responses to food images in women with bulimia versus anorexia nervosa. PLoS ONE 6, 1–8Crossref, Google Scholar
82 . (2008) Altered insula response to a taste stimulus in individuals recovered from restricting-type anorexia nervosa. Neuropsychopharmacology 33, 513–523Crossref, Google Scholar
83 . (2011) Effects of gustatory stimulation on brain activity during hunger and satiety in females with restricting-type anorexia nervosa: an fMRI study. J. Psychiatr. Res. 45, 395–403Crossref, Google Scholar
84 . (2009) Cortical activation in response to pure taste stimuli during the physiological states of hunger and satiety. Neuroimage 44, 1008–1021Crossref, Google Scholar
85 . (2008) Relation between obesity and blunted striatal response to foods is moderated by TaqIA A1 allele. Science 322, 449–452Crossref, Google Scholar
86 . (2012) Anorexia nervosa and obesity are associated with opposite brain reward response. Neuropsychopharmacology 37, 2031–2046Crossref, Google Scholar
87 . (2011) Increased neural processing of rewarding and aversive food stimuli in recovered anorexia nervosa. Biol. Psychiatry 70, 736–743Crossref, Google Scholar
88 . (2006) Anticipation of aversive visual stimuli is associated with increased insula activation in anxiety-prone subjects. Biol. Psychiatry 60, 402–409Crossref, Google Scholar
89 . (2006) An insular view of anxiety. Biol. Psychiatry 60, 383–387Crossref, Google Scholar
90 . (2000) Tracking the hemodynamic responses to reward and punishment in the striatum. J. Neurophysiol. 84, 3072–3077Crossref, Google Scholar
91 . (2009) Neural correlates of impaired cognitive–behavioral flexibility in anorexia nervosa. Am. J. Psychiatry 166, 608–616Crossref, Google Scholar
92 . (2011) Aberrant brain activation during a response inhibition task in adolescent eating disorder subtypes. Am. J. Psychiatry 168, 55–64Crossref, Google Scholar
93 . (2011) Demand-specific alteration of medial prefrontal cortex response during an inhibition task in recovered anorexic women. Int. J. Eat. Disord. 44, 1–8Crossref, Google Scholar
94 . (2003) Neurobiology of emotion perception II: implications for major psychiatric disorders. Biol. Psychiatry 54, 515–528Crossref, Google Scholar
95 . (2003) Neurobiology of emotion perception I: the neural basis of normal emotion perception. Biol. Psychiatry 54, 504–514Crossref, Google Scholar
96 . (2004) Neural coding of basic reward terms of animal learning theory, game theory, microeconomics and behavioural ecology. Science 14, 139–147Google Scholar
97 . (2006) The role of the basal ganglia in habit formation. Nat. Neurosci. Rev. 7, 464–476Crossref, Google Scholar
98 . (2002) Drug addiction and its underlying neurobiological basis: neuroimaging evidence for the involvement of the frontal cortex. Am. J. Psychiatry 159, 1642–1652Crossref, Google Scholar
99 . (2012) Addiction, compulsive drug seeking, and the role of frontostriatal mechanisms in regulating inhibitory control. Neurosci. Biobehav. Rev. 35, 248–275Crossref, Google Scholar
100 . (2010) Prefrontal–striatal pathway underlies cognitive regulation of craving. Proc. Natl. Acad. Sci. U.S.A. 107, 14811–14816Crossref, Google Scholar
101 . (2011) Sex differences precipitating anorexia nervosa in females: the estrogen paradox and a novel framework for targeting sex-specific neurocircuits and behavior. Curr. Top. Behav. Neurosci. 8, 189–207Crossref, Google Scholar
102 . (2010) The storm and stress of adolescence: insights from human imaging and mouse genetics. Dev. Psychobiol. 52, 225–235Google Scholar
103 . (2002) Reduced 5-HT2A receptor binding after recovery from anorexia nervosa. Biol. Psychiatry 52, 896–906Crossref, Google Scholar
104 . (2004) Altered 5-HT2A receptor binding after recovery from bulimia-type anorexia nervosa: relationships to harm avoidance and drive for thinness. Neuropsychopharmacology 29, 1143–1155Crossref, Google Scholar
105 . (2007) Exaggerated 5-HT1A but normal 5-HT2A receptor activity in individuals ill with anorexia nervosa. Biol. Psychiatry 61, 1090–1099Crossref, Google Scholar
106 . (2003) Decreased 5-HT2a receptor binding in patients with anorexia nervosa. J. Nucl. Med. 44, 163–169Google Scholar
107 . (2005) Altered brain serotonin 5-HT1A receptor binding after recovery from anorexia nervosa measured by positron emission tomography and [11C]WAY100635. Arch. Gen. Psychiatry 62, 1032–1041Crossref, Google Scholar
108 . (2008) Organic background of restrictive-type anorexia nervosa suggested by increased serotonin1A receptor binding in right frontotemporal cortex of both lean and recovered patients: [18F]MPPF PET scan study. Biol. Psychiatry 64, 1009–1013Crossref, Google Scholar
109 . (2011) Opponency revisited: competition and cooperation between dopamine and serotonin. Neuropsychopharmacol. Rev. 36, 74–97Crossref, Google Scholar
110 . (1992) Promises and problems with the circumplex model of emotion. In Emotion (Review of Personality and Social Psychology, Vol. 13) (Clark M, ed.), pp. 25–29, SageGoogle Scholar
111 . (2008) Anticipatory affect: neural correlates and consequences for choice. Philos. Trans. R. Soc. Lond. B: Biol. Sci. 363, 3771–3786Crossref, Google Scholar
112 . (1999) The two general activation systems of affect: structural findings, evolutionary considerations, and psychobiological evidence. J. Pers. Soc. Psychol. 76, 820–838Crossref, Google Scholar
113 . (2007) Adolescent anorexia nervosa: cross-sectional and follow-up frontal gray matter disturbances detected with proton magnetic resonance spectroscopy. J. Psychiatr. Res. 41, 952–958Crossref, Google Scholar
114 . (2012) Grey matter abnormalities within cortico–limbic–striatal circuits in acute and weight-restored anorexia nervosa patients. Neuroimage 59, 1106–1113Crossref, Google Scholar
115 . (2012) Structural magnetic resonance imaging in eating disorders: a systematic review of voxel-based morphometry studies. Eur. Eat. Disord. Rev. 20, 94–105Crossref, Google Scholar
116 . (2012) Structural brain abnormalities in adolescent anorexia nervosa before and after weight recovery and associated hormonal changes. Psychosom. Med. 74, 574–582Crossref, Google Scholar
117 . (2012) Metabolic gray matter changes of adolescents with anorexia nervosa in combined MR proton and phosphorus spectroscopy. Neuroradiology 54, 753–764Crossref, Google Scholar
118 . (2012) Animal models of sugar and fat bingeing: relationship to food addiction and increased body weight. Methods Mol. Biol. 829, 351–365Crossref, Google Scholar
119 . (2010) Dopamine D2 receptors in addiction-like reward dysfunction and compulsive eating in obese rats. Nat. Neurosci. 13, 635–641Crossref, Google Scholar
120 . (2002) Longitudinal comparison of anorexia nervosa subtypes. Int. J. Eat. Disord. 31, 191–201Crossref, Google Scholar
121 . (2012) A debate on current eating disorder diagnoses in light of neurobiological findings: is it time for a spectrum model? BMC Psychiatry 12, 76Crossref, Google Scholar
122 . (2012) Neural basis of a multidimensional model of body image distortion in anorexia nervosa. Neurosci. Biobehav. Rev. 36, 1839–1847Crossref, Google Scholar
123 . (2012) Diminished size-weight illusion in anorexia nervosa: evidence for visuo-proprioceptive integration deficit. Exp. Brain Res. 217, 79–87Crossref, Google Scholar